As you step outside on a sunny day, have you ever wondered how plants are able to harness the power of sunlight to produce energy? The process they use is called photosynthesis, and it is nothing short of remarkable.
Through a series of intricate steps, plants and other photosynthetic organisms transform light energy into chemical energy, fueling the synthesis of vital biomolecules. But how exactly does photosynthesis work? What is the equation behind it? And what are the specific steps involved?
In this discussion, we will unravel the mysteries of photosynthesis, exploring its definition, equation, steps, and overall process. Prepare to be amazed as we delve into the fascinating world of photosynthesis and discover its crucial role in sustaining life on our planet.
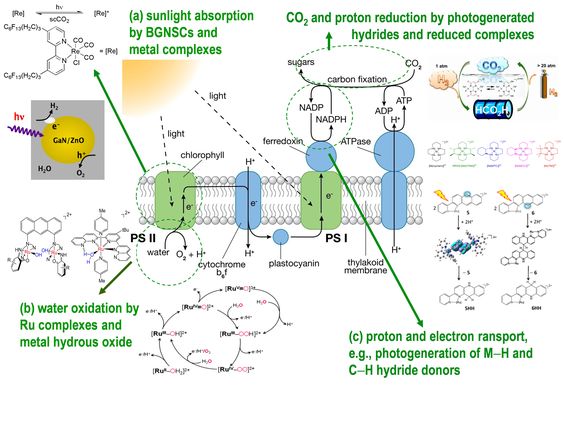
Photosynthesis: An Overview
Photosynthesis, an intricate process that converts electromagnetic radiation into chemical energy, is essential for the survival and growth of photosynthetic organisms. It’s a fundamental process that sustains life on Earth by converting light energy into usable chemical energy in the form of carbohydrates.
Through photosynthesis, plants, algae, and some bacteria are able to synthesize their own food and produce oxygen as a byproduct. This process begins with the absorption of light by chlorophylls and other pigments, which transfer the energy to the reaction center. The absorbed light energy is then used to drive a series of chemical reactions that convert carbon dioxide and water into glucose and oxygen.
This transformation occurs in two stages: the light-dependent reactions, which take place in the thylakoid membranes and generate ATP and NADPH, and the Calvin cycle, which occurs in the stroma and uses the energy from ATP and NADPH to fix carbon dioxide and produce glucose.
Photosynthesis is influenced by various factors, including light intensity, carbon dioxide concentration, and temperature. Understanding the intricacies of photosynthesis is crucial in our pursuit of sustainable energy sources and in our appreciation of the interconnectedness of all life forms on our planet.
The Equations of Photosynthesis
Now let’s explore the equations of photosynthesis.
Photosynthesis involves the conversion of carbon dioxide and water into carbohydrates and oxygen.
This process is facilitated by photosynthetic pigments, such as chlorophylls, carotenoids, and pheophytin, which absorb electromagnetic radiation and transfer energy to the reaction center.
Understanding these equations and the role of pigments is crucial in comprehending the intricate process of photosynthesis.
Photosynthetic Equations
The process of photosynthesis can be represented by a series of equations that describe the conversion of light energy into chemical energy in plants and other photosynthetic organisms.
The main equation for photosynthesis is:
6CO2 + 6H2O + light energy → C6H12O6 + 6O2
In this equation, carbon dioxide (CO2) and water (H2O) are converted into glucose (C6H12O6) and oxygen (O2) in the presence of light energy. This equation summarizes the overall process of photosynthesis.
Additionally, there are several other equations that represent the specific reactions occurring during photosynthesis.
For example, the light-dependent reactions can be represented by the following equation:
H2O + light energy → ATP + NADPH + O2
In this equation, water is split into oxygen, ATP (adenosine triphosphate), and NADPH (nicotinamide adenine dinucleotide phosphate) using light energy.
Pigments and Absorption
Light-absorbing pigments play a crucial role in the process of photosynthesis, capturing electromagnetic radiation and transferring energy to drive the chemical reactions that convert carbon dioxide and water into carbohydrates and oxygen.
These pigments, such as chlorophylls, carotenoids, and pheophytin, are located in the chloroplasts of plants. Chlorophyll is the primary photoreceptor in green plants, with its network of alternating single and double bonds allowing it to effectively absorb light in the visible region of the spectrum.
Bacteriorhodopsin, found in halobacteria, absorbs light photons and triggers a conformational change in the protein, resulting in the expulsion of protons from the cell. Cyanobacteria and red algae use phycobilins, covalently linked to specific binding proteins, as light-harvesting pigments.
Carotenoids, found in thylakoid membranes, serve as secondary light-absorbing pigments, absorbing light at wavelengths not absorbed by chlorophylls. These pigments, through their absorption of light energy, facilitate the process of photosynthesis.
Photosynthetic Pigments and Their Functions
Now let’s explore the fascinating world of photosynthetic pigments and their functions. These pigments play a crucial role in capturing light energy during photosynthesis.
By absorbing specific wavelengths of light, they transfer this energy to the reaction center, where it’s converted into chemical energy.
Chlorophylls, carotenoids, and pheophytin are some of the important photosynthetic pigments that contribute to the efficient capture of light and the overall process of photosynthesis.
Pigment Absorption Spectra
With a focus on photosynthetic pigments and their functions, let’s delve into the fascinating world of pigment absorption spectra.
Pigment absorption spectra refer to the range of wavelengths of light that different photosynthetic pigments can absorb. Chlorophylls, the primary pigments in plants, have absorption peaks in the red and blue regions of the spectrum, with minimal absorption in the green region, giving plants their characteristic green color.
Carotenoids, another group of pigments, have absorption peaks in the blue and green regions, contributing to the yellow, orange, and red colors observed in fruits and flowers. By having different absorption spectra, these pigments allow plants to efficiently capture light energy from different parts of the spectrum, maximizing their ability to carry out photosynthesis.
Understanding pigment absorption spectra is crucial in unraveling the intricate mechanisms of photosynthesis.
Role in Light Capture
Continuing our exploration of pigment absorption spectra, we now turn our attention to the crucial role that photosynthetic pigments play in capturing light for the process of photosynthesis.
Photosynthetic pigments, such as chlorophylls, carotenoids, and pheophytin, are responsible for absorbing light energy and transferring it to the reaction center. Chlorophyll, the principal photoreceptor in green plants, has a network of alternating single and double bonds that make it an effective photoreceptor. It has solid absorption bands in the visible region of the spectrum.
Carotenoids, found in thylakoid membranes, serve as secondary light-absorbing pigments and absorb light at wavelengths not absorbed by chlorophylls.
The photosynthetic pigments in plants and other organisms are essential for capturing light energy and initiating the process of photosynthesis.
Factors Influencing Photosynthesis
Factors influencing photosynthesis include:
- Light intensity: Increased light intensity leads to higher rates of light-dependent reactions and overall photosynthesis. Different photosynthetic systems absorb light energy more effectively at different wavelengths, affecting the efficiency of photosynthesis.
- Carbon dioxide concentration: Higher carbon dioxide concentration in the atmosphere increases the rate of carbon incorporation into carbohydrates, up to a certain point. This is because the enzyme responsible for carbon fixation, Rubisco, becomes saturated with carbon dioxide at high concentrations.
- Temperature: Enzymes involved in photosynthesis have an optimum temperature at which they function most efficiently. At higher temperatures, enzymes denature and their activity decreases, negatively impacting photosynthesis. Conversely, at lower temperatures, enzyme activity slows down, limiting the rate of photosynthesis.
Understanding these factors is crucial for optimizing photosynthesis in agricultural practices and managing the impacts of climate change on plant productivity.
The Process of Photosynthesis
Light intensity, carbon dioxide concentration, and temperature are crucial factors that influence the process of photosynthesis in plants.
Light intensity determines the amount of energy available for photosynthesis. As light intensity increases, the rate of photosynthesis also increases, up to a certain point where the rate levels off due to other limiting factors.
Carbon dioxide concentration affects the rate of carbon incorporation into carbohydrates. When carbon dioxide concentration increases, the rate of photosynthesis also increases, as long as other factors like light and temperature aren’t limiting.
Temperature affects the rate of the light-independent reactions catalyzed by enzymes. Enzymes involved in photosynthesis have an optimum temperature at which they function most efficiently. Below or above this optimum temperature, the rate of photosynthesis decreases.
Therefore, maintaining suitable light intensity, carbon dioxide concentration, and temperature is crucial for maximizing the process of photosynthesis in plants.
The Steps of Photosynthesis
The process of photosynthesis can be divided into several distinct steps, each playing a crucial role in converting light energy into chemical energy and producing carbohydrates.
The first step is the absorption of light by chlorophylls in chloroplasts. This light energy excites electrons in the chlorophyll molecules, initiating the process. Electron transfer then occurs through a chain of electron transfer molecules, which leads to the generation of ATP from the movement of protons across the membrane. This ATP serves as the energy source for the subsequent steps.
The next step is carbon fixation, where carbon dioxide is reduced into six-carbon sugar molecules. This process requires the enzyme Rubisco and is critical for the production of carbohydrates.
Photosynthesis is divided into two stages: the light-dependent reactions and the Calvin cycle. The light-dependent reactions take place in the thylakoid membrane and use light energy to make ATP and NADPH. The Calvin cycle, which takes place in the stroma, uses energy from ATP and NADPH to produce glyceraldehyde 3-phosphate (GA3P) from carbon dioxide.
These steps of photosynthesis are vital for the survival and growth of plants, as they provide the energy and building blocks necessary for the synthesis of carbohydrates and other biomolecules.
Light-Dependent Reactions
As we explore the intricate process of photosynthesis, we now turn our focus to the essential phase known as the light-dependent reactions. These reactions occur in the thylakoid membrane of the chloroplasts and play a crucial role in capturing and converting light energy into chemical energy. The main purpose of these reactions is to produce energy-rich molecules such as ATP and NADPH, which are used in the subsequent steps of photosynthesis.
The light-dependent reactions involve two key components: Photosystem II (PSII) and Photosystem I (PSI). PSII absorbs photons of light and uses the energy to split water molecules, releasing oxygen as a byproduct. This process, known as photolysis, replenishes the electrons lost by PSII. The excited electrons then pass through an electron transport chain, generating a proton gradient across the thylakoid membrane. This proton gradient drives the synthesis of ATP through a process called chemiosmosis.
Meanwhile, PSI absorbs light energy and uses it to re-energize electrons. These energized electrons are transferred to another electron transport chain, ultimately reducing NADP+ to NADPH. NADPH serves as a high-energy electron carrier in the Calvin cycle, which is the next phase of photosynthesis.
The Calvin Cycle
Now let’s explore the intricate process of the Calvin Cycle.
In this essential phase of photosynthesis, carbon fixation occurs, where carbon dioxide is converted into six-carbon sugar molecules.
The enzyme Rubisco plays a critical role in this step by facilitating the fixation of CO2 into 3-phosphoglycerate.
Understanding the Calvin Cycle is crucial to comprehending the overall mechanism of photosynthesis and the formation of glucose in plants.
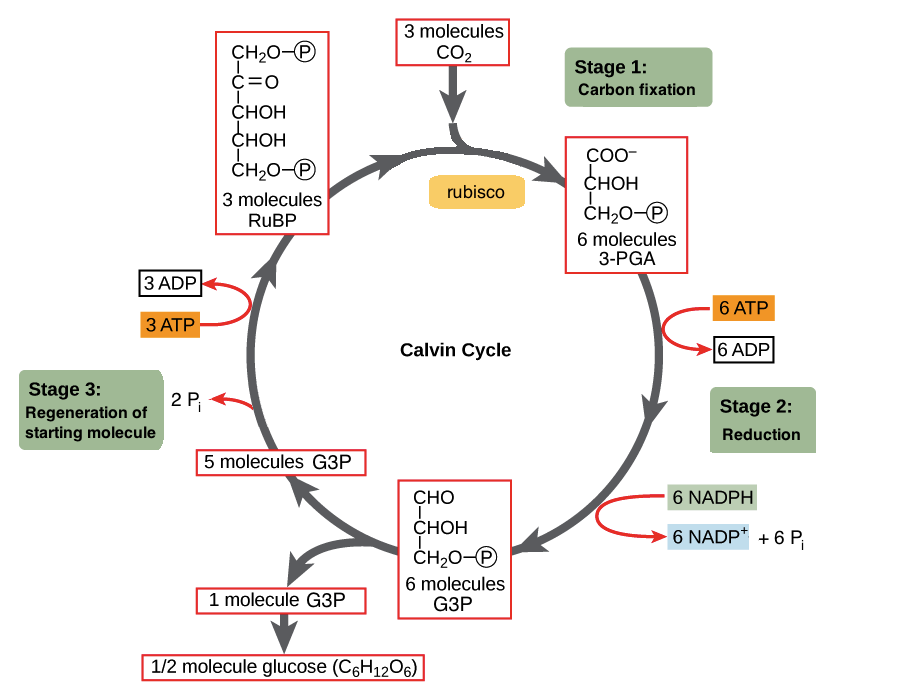
Carbon Fixation Process
The Carbon Fixation Process, also known as the Calvin Cycle, is a fundamental metabolic pathway in photosynthesis, responsible for incorporating carbon dioxide into organic molecules. This process occurs in the stroma of the chloroplasts.
The first step in the Calvin Cycle is the fixation of carbon dioxide, which is catalyzed by the enzyme Rubisco. Carbon dioxide reacts with a five-carbon sugar, ribulose-1,5-bisphosphate (RuBP), resulting in the formation of two molecules of a three-carbon compound, 3-phosphoglycerate (3-PGA).
In the second step, ATP and NADPH produced during the light-dependent reactions provide the energy and reducing power to convert 3-PGA into glyceraldehyde-3-phosphate (GA3P).
Finally, in the third step, some of the GA3P is used to regenerate RuBP, while the rest is used to produce glucose and other organic molecules.
The Calvin Cycle is a complex and precise process that plays a crucial role in the production of carbohydrates, which serve as energy sources and building blocks in plants.
Formation of GA3P
During the Calvin Cycle, glyceraldehyde-3-phosphate (GA3P) is formed as a key intermediate in the production of carbohydrates in photosynthetic organisms. The Calvin Cycle, also known as the light-independent reactions, takes place in the stroma of the chloroplasts.
This cycle uses energy from ATP and NADPH, which are produced during the light-dependent reactions, to convert carbon dioxide (CO2) into GA3P. The first step of the Calvin Cycle is the fixation of CO2, where the enzyme Rubisco catalyzes the reaction between CO2 and ribulose 1,5-biphosphate. This forms an unstable six-carbon molecule, which quickly breaks down into two molecules of 3-phosphoglycerate.
Through a series of enzymatic reactions, 3-phosphoglycerate is converted into GA3P. GA3P is then used to synthesize glucose and other carbohydrates, providing the necessary energy and carbon molecules for the growth and development of photosynthetic organisms.
Role of Rubisco
As we continue our exploration of photosynthesis and the formation of glyceraldehyde-3-phosphate (GA3P), let’s now delve into the pivotal role of Rubisco, the enzyme responsible for the fixation of carbon dioxide in the Calvin Cycle.
Rubisco, short for ribulose-1,5-bisphosphate carboxylase/oxygenase, is the most abundant enzyme on Earth. It catalyzes the first step of the Calvin Cycle, which is the fixation of carbon dioxide into an organic molecule.
Rubisco combines carbon dioxide with a five-carbon sugar called ribulose-1,5-bisphosphate (RuBP) to form a six-carbon molecule that’s highly unstable. This molecule then breaks down into two molecules of 3-phosphoglycerate (3-PGA), which is further converted into glyceraldehyde-3-phosphate (GA3P).
Rubisco plays a crucial role in carbon fixation and is essential for the production of organic compounds in photosynthetic organisms.
Photosynthesis in Sulfur Bacteria
Photosynthesis in sulfur bacteria involves the utilization of hydrogen sulfide as a substitute for water in the light-dependent reactions. These bacteria have adapted to live in environments with high sulfur concentrations, such as hot springs and deep-sea hydrothermal vents. Instead of using water as an electron donor, sulfur bacteria use hydrogen sulfide (H2S), which is abundant in these environments.
The process of photosynthesis in sulfur bacteria is similar to that in plants, but with different reactants. The light-dependent reactions occur in specialized structures called chromatophores, which contain photosynthetic pigments like chlorophyll or carotenoids. These pigments absorb light energy and transfer it to reaction centers, where electron transfer takes place. This generates ATP and reducing equivalents such as NADPH.
The products of photosynthesis in sulfur bacteria include carbohydrates, sulfur gas, and water molecules. The carbohydrates serve as a source of energy for the bacteria, while the sulfur gas is released into the environment. The water molecules are formed as a byproduct of the light-dependent reactions.
Photosynthesis in sulfur bacteria plays an important role in the sulfur cycle, as it contributes to the production of organic compounds and the release of sulfur gas into the environment. Understanding this unique form of photosynthesis can provide insights into the diverse strategies that organisms have evolved to harness light energy for their survival.
The Importance of Photosynthesis
In the realm of photosynthesis, the significance of this process can’t be overstated. Photosynthesis is the primary source of energy for autotrophs, enabling them to produce their own food. Through the conversion of electromagnetic radiation into chemical energy, photosynthesis plays a crucial role in maintaining oxygen levels in the atmosphere. The products of photosynthesis, such as carbohydrates, contribute to the carbon cycle in various ecosystems, providing energy transfer within the food chain and serving as carbon molecules for biomolecule synthesis.
Heterotrophs, including animals and humans, rely on photosynthesis indirectly for their energy by consuming autotrophs. The symbiotic relationship between plants, animals, and humans is dependent on photosynthesis. Plants produce oxygen as a byproduct of photosynthesis, which is vital for aerobic respiration in animals and humans. In addition, photosynthesis provides the necessary organic compounds for the growth and development of all organisms.
Furthermore, photosynthesis has a significant impact on the environment. It helps regulate the Earth’s climate by reducing the amount of carbon dioxide in the atmosphere and storing it in the form of carbohydrates. This process plays a crucial role in mitigating climate change by acting as a natural carbon sink.
Conclusion
In conclusion, photosynthesis is a complex and vital process that allows plants and other photosynthetic organisms to convert light energy into chemical energy.
Through the use of pigments such as chlorophylls, carotenoids, and pheophytin, these organisms are able to absorb light and carry out the necessary reactions to produce glucose and oxygen.
Factors such as light intensity, carbon dioxide concentration, and temperature play crucial roles in the efficiency of photosynthesis.
By understanding the intricacies of this process, we can better appreciate its significance in sustaining life on Earth.
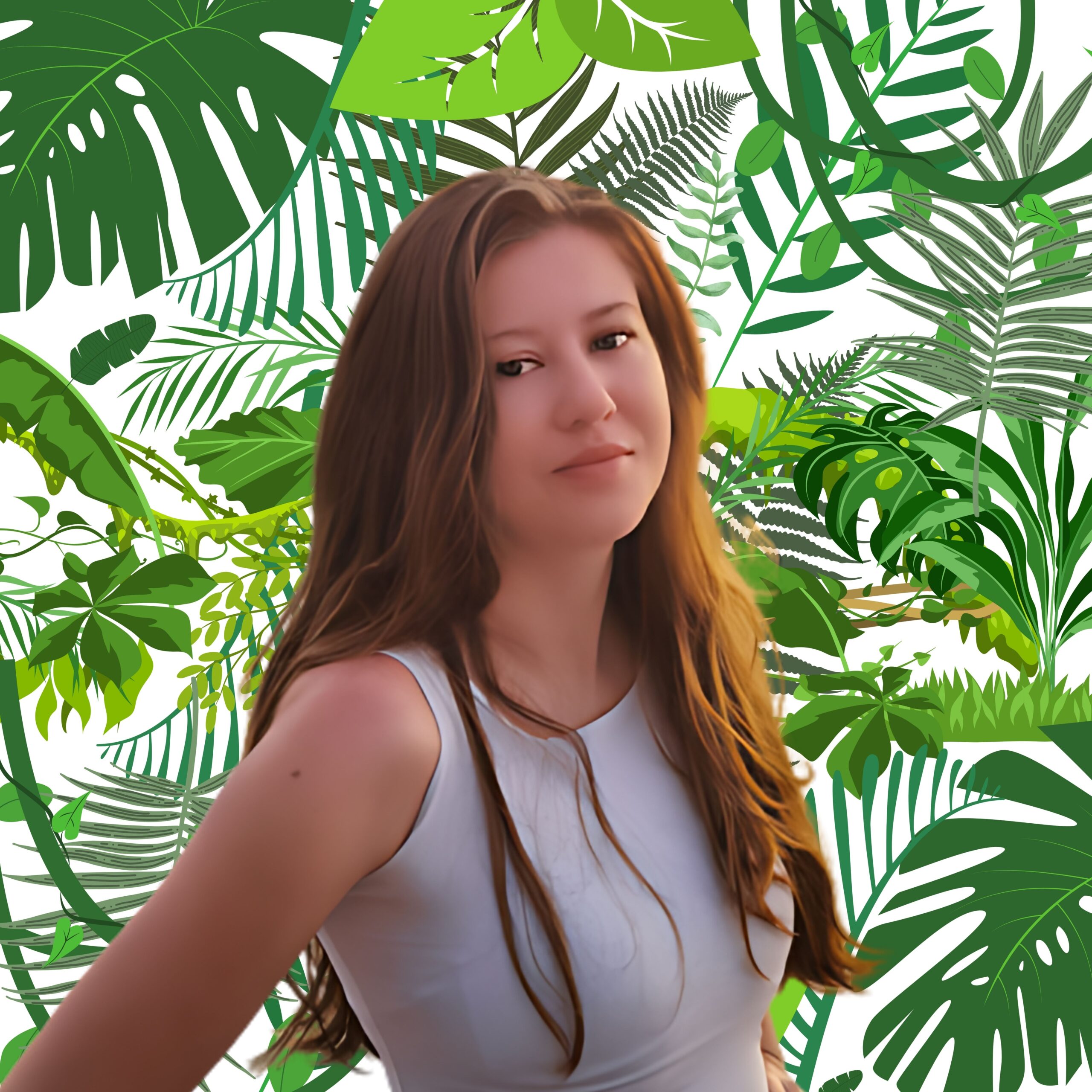
Erzsebet Frey (Eli Frey) is an ecologist and online entrepreneur with a Master of Science in Ecology from the University of Belgrade. Originally from Serbia, she has lived in Sri Lanka since 2017. Eli has worked internationally in countries like Oman, Brazil, Germany, and Sri Lanka. In 2018, she expanded into SEO and blogging, completing courses from UC Davis and Edinburgh. Eli has founded multiple websites focused on biology, ecology, environmental science, sustainable and simple living, and outdoor activities. She enjoys creating nature and simple living videos on YouTube and participates in speleology, diving, and hiking.