Brace yourself for a journey through the intricate pathways of energy production, as we explore the location, enzymes, steps, products, and even a diagram of this vital cycle.
From the mitochondria of eukaryotic cells to the cytoplasm of prokaryotes, the Krebs cycle takes place in different cellular compartments. Enzymes play a crucial role in facilitating the chemical reactions, generating high-energy molecules like NADH and FADH2, and ultimately producing ATP.
But what is the purpose of the Krebs cycle? How does it depend on oxygen? And what factors can inhibit its function? Get ready to dive deep into the fascinating world of the Krebs cycle and uncover the answers to these questions and more.
Krebs Cycle Location
The Krebs Cycle is primarily located in the mitochondria of eukaryotic cells and in the cytoplasm of prokaryotic cells. In eukaryotes, the enzymes of the Krebs Cycle are situated in the inner membrane or matrix space of the mitochondria. However, in prokaryotes, these enzymes are present in the cytoplasm. Except for succinate dehydrogenase and aconitase, all the enzymes involved in the Krebs Cycle are found in the matrix of the mitochondria.
The Krebs Cycle consists of several steps. First, acetyl CoA condenses with oxaloacetate to form citrate. Then, citrate is isomerized into isocitrate. Isocitrate undergoes oxidative decarboxylation to form α-ketoglutarate. α-ketoglutarate is then oxidatively decarboxylated to form succinyl-CoA. Succinyl-CoA is converted into succinate, followed by the hydration of fumarate to malate. Finally, L-malate is dehydrogenated to oxaloacetate.
The Krebs Cycle produces various products, including CO2, NADH, FADH2, and ATP. These high-energy molecules are essential for ATP production in the electron transport chain. At each turn of the cycle, three molecules of NADH, one molecule of FADH2, one molecule of GTP (or ATP), and two molecules of CO2 are generated. Moreover, oxaloacetate is formed at the end of the cycle.
To summarize, the Krebs Cycle is located in the mitochondria of eukaryotic cells and the cytoplasm of prokaryotic cells. It involves a series of enzymatic reactions that result in the production of CO2, NADH, FADH2, and ATP. These products serve as crucial sources of energy for cellular processes.
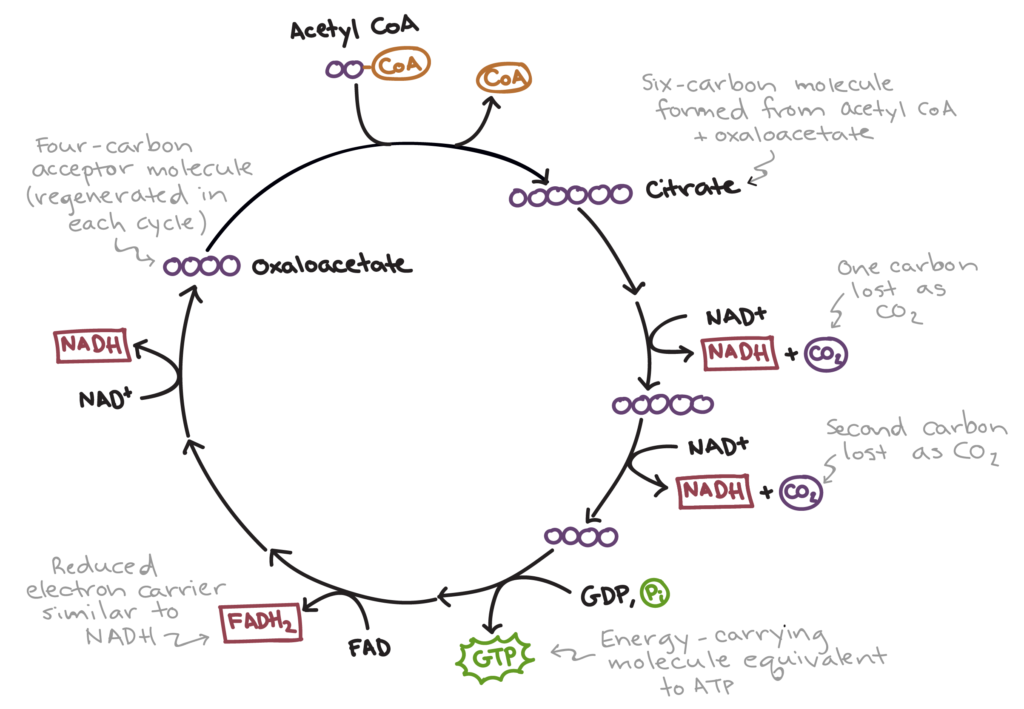
Enzymes Involved
Located in the matrix of the mitochondria and cytoplasm, the Krebs Cycle involves a series of enzymatic reactions that play a crucial role in cellular energy production. There are several enzymes involved in this cycle, each with specific functions.
The first enzyme, citrate synthase, catalyzes the condensation of acetyl CoA with oxaloacetate to form citrate. Aconitase then isomerizes citrate into isocitrate. Next, isocitrate dehydrogenase oxidatively decarboxylates isocitrate to form α-ketoglutarate. α-ketoglutarate dehydrogenase further oxidatively decarboxylates α-ketoglutarate to form succinyl-CoA. Succinyl-CoA synthetase then converts succinyl-CoA into succinate. Succinate dehydrogenase catalyzes the oxidation of succinate to fumarate. Fumarase hydrates fumarate to malate, which is then dehydrogenated by malate dehydrogenase to form oxaloacetate.
These enzymatic reactions result in the production of high-energy molecules, including NADH, FADH2, and ATP. These molecules are essential for the electron transport chain, where they contribute to the production of ATP through oxidative phosphorylation.
The Krebs Cycle also produces CO2 as a byproduct of the decarboxylation reactions.
Krebs Cycle Steps
To initiate the Krebs Cycle, acetyl CoA and oxaloacetate undergo condensation to form citrate. This step is catalyzed by the enzyme citrate synthase.
Next, citrate is isomerized into isocitrate with the help of the enzyme aconitase. Isocitrate is then oxidatively decarboxylated by isocitrate dehydrogenase, resulting in the formation of α-ketoglutarate.
The next step involves the oxidative decarboxylation of α-ketoglutarate to form succinyl-CoA, which is catalyzed by the enzyme α-ketoglutarate dehydrogenase. Succinyl-CoA is then converted into succinate by succinyl-CoA synthetase.
Fumarase then hydrates fumarate to form malate. Finally, malate is dehydrogenated by malate dehydrogenase to regenerate oxaloacetate, completing one turn of the Krebs Cycle.
Each turn of the Krebs Cycle produces 3 molecules of NADH, 1 molecule of FADH2, 1 molecule of GTP (or ATP), and 2 molecules of CO2. These products play a crucial role in providing high-energy molecules to the electron transport chain for ATP production. Oxaloacetate is also formed at the end of the cycle, ready to initiate another round of the Krebs Cycle.
The Krebs Cycle is essential for the complete oxidation of glucose and the generation of energy-rich molecules. It takes place in the mitochondria of eukaryotic cells and the cytoplasm of prokaryotic cells. The cycle requires oxygen and is inhibited by factors such as the absence of oxygen, low levels of oxaloacetate or pyruvate, the absence of necessary enzymes and coenzymes, high levels of ATP and NADH, and the accumulation of ketone bodies.
Krebs Cycle Products
Now let’s discuss the important points regarding Krebs Cycle Products.
The Krebs Cycle produces energy-rich molecules such as NADH, FADH2, and ATP. These molecules play a crucial role in providing high-energy electrons to the electron transport chain for the production of ATP.
Understanding the products of the Krebs Cycle is essential in grasping the overall energy production process in cellular respiration.
Energy-rich Molecules
Energy-rich molecules, including NADH, FADH2, and ATP, are produced during the Krebs cycle in order to provide high-energy compounds for ATP production in the electron transport chain.
NADH and FADH2 are electron carriers that store energy through the reduction of NAD+ and FAD, respectively. These molecules are generated through a series of redox reactions during the cycle.
NADH and FADH2 then transfer their electrons to the electron transport chain, where ATP is synthesized through oxidative phosphorylation.
ATP, on the other hand, is a nucleotide that serves as the primary energy source for cellular processes. It’s produced through substrate-level phosphorylation during the conversion of succinyl-CoA to succinate in the Krebs cycle.
Electron Transport Chain
The Electron Transport Chain is a crucial step in the Krebs Cycle. It occurs in the inner membrane of the mitochondria in eukaryotes. During this process, high-energy molecules like NADH and FADH2 transfer their electrons to generate ATP through oxidative phosphorylation.
The electrons from NADH and FADH2 are passed through a series of protein complexes in the Electron Transport Chain. This creates a flow of electrons that drives the synthesis of ATP. As the electrons move through the chain, they release energy. This energy is used to pump protons across the inner membrane, creating an electrochemical gradient.
The electrochemical gradient is then used by ATP synthase to generate ATP. In addition to ATP, the Electron Transport Chain also produces water as a byproduct of the final electron acceptor, oxygen.
Krebs Cycle Diagram
A Krebs Cycle diagram illustrates the sequential steps and key molecules involved in one complete turn of the cycle. It provides a visual representation of the metabolic pathway, making it easier to understand the complex series of reactions.
The diagram typically starts with acetyl CoA and oxaloacetate, which condense to form citrate. Through a series of enzymatic reactions, citrate is isomerized to isocitrate, followed by oxidative decarboxylation to form α-ketoglutarate. Further oxidative decarboxylation leads to the formation of succinyl-CoA, which is then converted into succinate. Hydration of fumarate produces malate, and subsequent dehydrogenation of malate forms oxaloacetate, completing the cycle.
The diagram also highlights the key molecules produced at each step, including CO2, NADH, FADH2, and ATP. These high-energy molecules play a crucial role in the electron transport chain, contributing to ATP production.
Eukaryotic Mitochondrial Location
In eukaryotes, the Krebs cycle takes place in the mitochondria, specifically in the inner membrane or matrix space. The enzymes involved in the Krebs cycle are located in these regions, with the exception of succinate dehydrogenase and aconitase, which are present in the matrix.
This localization is crucial for energy production and plays a vital role in cellular respiration.
Mitochondrial Enzyme Localization
Occasionally, eukaryotic mitochondrial enzymes are localized in the inner membrane or matrix space of the mitochondria. These enzymes play crucial roles in the Krebs cycle, also known as the citric acid cycle or tricarboxylic acid cycle.
The inner membrane of the mitochondria houses enzymes such as succinate dehydrogenase and aconitase, while the matrix contains enzymes like citrate synthase, isocitrate dehydrogenase, α-ketoglutarate dehydrogenase, succinyl-CoA synthetase, fumarase, and malate dehydrogenase.
The localization of these enzymes in specific compartments allows for efficient substrate channeling and regulation of metabolic pathways. This compartmentalization ensures that the intermediates and products of the Krebs cycle are efficiently processed and utilized for ATP production.
Understanding the localization of these enzymes is crucial for studying the regulation and functioning of the Krebs cycle in eukaryotic mitochondria.
Role in Energy Production
The role of the Krebs cycle in energy production is essential, as it takes place within the mitochondria of eukaryotic cells. This cycle plays a crucial role in the production of high-energy molecules that are required for ATP production.
During the Krebs cycle, acetyl CoA is condensed with oxaloacetate to form citrate. Subsequent steps involve isomerization, oxidative decarboxylation, conversion, hydration, and dehydrogenation reactions, resulting in the production of CO2, NADH, FADH2, and ATP.
These energy-rich molecules are then used by the electron transport chain to generate ATP. The Krebs cycle occurs in the matrix of the mitochondria and is inhibited by factors such as the absence of oxygen, low levels of oxaloacetate or pyruvate, high levels of ATP and NADH, and the accumulation of ketone bodies.
Importance in Cellular Respiration
The eukaryotic mitochondrial location of the Krebs cycle is crucial for its role in cellular respiration. Within the mitochondria, this cycle takes place in the matrix, where important enzymes are located. The enzymes involved in the Krebs cycle, such as citrate synthase, aconitase, and isocitrate dehydrogenase, are present in the matrix except for succinate dehydrogenase and aconitase, which are found in the inner membrane.
This specific location allows for efficient coordination and regulation of the cycle. The Krebs cycle plays a significant role in cellular respiration by providing high-energy molecules, including NADH, FADH2, and ATP, to the electron transport chain for ATP production. Without the eukaryotic mitochondrial location, the Krebs cycle wouldn’t be able to function effectively in generating energy for the cell.
Prokaryotic Cytoplasmic Location
Located within the cytoplasm of prokaryotic cells, the Krebs cycle serves as a key metabolic pathway for energy production. In prokaryotes, the Krebs cycle takes place directly in the cytoplasm. Unlike eukaryotes, prokaryotes lack mitochondria, so the enzymes involved in the Krebs cycle aren’t located in the inner membrane or matrix space.
Instead, these enzymes are present in the cytoplasm of prokaryotic cells, specifically in the cytoplasmic matrix. The enzymes involved in the Krebs cycle in prokaryotes include citrate synthase, aconitase, isocitrate dehydrogenase, α-ketoglutarate dehydrogenase, succinyl-CoA synthetase, succinate dehydrogenase, fumarase, and malate dehydrogenase.
The Krebs cycle in prokaryotes follows the same steps as in eukaryotes. These steps include the condensation of acetyl CoA with oxaloacetate to form citrate, the isomerization of citrate into isocitrate, the oxidative decarboxylation of isocitrate to form α-ketoglutarate, and the subsequent oxidative decarboxylation of α-ketoglutarate to form succinyl-CoA. The cycle continues with the conversion of succinyl-CoA into succinate, the hydration of fumarate to malate, and the dehydrogenation of L-malate to oxaloacetate.
In prokaryotic cells, the Krebs cycle produces key products such as CO2, NADH, FADH2, and ATP. These high-energy molecules are then utilized by the electron transport chain to generate ATP. Overall, the Krebs cycle plays a crucial role in energy production within the cytoplasm of prokaryotic cells.
Inhibition of the Krebs Cycle
Inhibition of the Krebs Cycle occurs due to various factors. One factor is the absence of oxygen, which is required for the proper functioning of the cycle. When oxygen isn’t available, the Krebs Cycle can’t proceed. Another factor is low levels of key metabolites such as oxaloacetate or pyruvate, which are important for the cycle’s progression. The absence of necessary enzymes and coenzymes can also hinder the cycle’s functioning. High levels of ATP and NADH, which are energy-rich molecules produced by the cycle, can act as feedback inhibitors. They inhibit specific enzymes involved in the cycle. Lastly, the accumulation of ketone bodies can inhibit the Krebs Cycle. Ketone bodies are produced during periods of prolonged fasting or uncontrolled diabetes. These inhibitory factors disrupt the normal flow of the cycle and ultimately affect the production of ATP, which is the main purpose of the Krebs Cycle.
Factors That Inhibit the Krebs Cycle
When inhibitory factors disrupt the normal flow of the Krebs Cycle, the production of ATP, which is the main purpose of the cycle, is affected. Several factors can inhibit the Krebs Cycle, leading to a decrease in ATP production.
One such factor is the absence of oxygen. Without oxygen, the Krebs Cycle can’t proceed as it relies on the availability of molecular oxygen to regenerate important coenzymes like NAD and FAD.
Additionally, low levels of oxaloacetate or pyruvate can also inhibit the cycle. These molecules are necessary for the initial steps of the cycle and their scarcity can disrupt its progression.
Another inhibitory factor is the absence of necessary enzymes and coenzymes. The Krebs Cycle relies on specific enzymes and coenzymes for each step, and their absence can halt the cycle.
High levels of ATP and NADH, which are the energy-rich molecules produced by the cycle, can also inhibit its progression.
Finally, the accumulation of ketone bodies can disrupt the Krebs Cycle. Ketone bodies can interfere with the enzymes involved in the cycle, leading to a decrease in ATP production.
Understanding these inhibitory factors is crucial for maintaining the normal functioning of the Krebs Cycle and ensuring adequate ATP production.
Krebs Cycle Reactions in Cells
Now let’s delve into the reactions of the Krebs Cycle in cells.
This metabolic pathway plays a crucial role in cellular energy production. It takes place in the mitochondria of eukaryotic cells and the cytoplasm of prokaryotic cells.
The Krebs Cycle involves a series of enzymatic reactions that result in the production of high-energy molecules, such as NADH, FADH2, and ATP, which are essential for ATP production in the electron transport chain.
Cellular Energy Production
Cellular energy production is facilitated by the Krebs cycle, a series of enzymatic reactions that occur in the mitochondria of eukaryotic cells and the cytoplasm of prokaryotic cells. The Krebs cycle, also known as the citric acid cycle or the tricarboxylic acid cycle, plays a crucial role in generating high-energy molecules for ATP production.
It begins with the condensation of acetyl CoA with oxaloacetate to form citrate and proceeds through a series of steps that involve isomerization, oxidative decarboxylation, conversion, hydration, and dehydrogenation reactions. Throughout the cycle, CO2, NADH, FADH2, and ATP are produced.
The Krebs cycle requires oxygen and is inhibited by factors such as low levels of oxaloacetate or pyruvate, absence of necessary enzymes and coenzymes, high levels of ATP and NADH, and accumulation of ketone bodies.
Metabolic Pathway Overview
The Krebs cycle, also known as the citric acid cycle or the tricarboxylic acid cycle, plays a crucial role in cellular energy production by generating high-energy molecules for ATP production.
This metabolic pathway occurs in the mitochondria of eukaryotic cells and in the cytoplasm of prokaryotic cells. The enzymes involved in the Krebs cycle are located in the inner membrane or matrix space of mitochondria, except for succinate dehydrogenase and aconitase, which are present in the matrix.
The cycle consists of multiple steps, including the condensation of acetyl CoA with oxaloacetate, the isomerization of citrate into isocitrate, and the oxidative decarboxylation of α-ketoglutarate to form succinyl-CoA.
The products of the Krebs cycle include CO2, NADH, FADH2, and ATP, which are used as high-energy molecules in the electron transport chain for ATP production.
Location and Regulation
The Krebs cycle reactions occur within specific cellular compartments and are tightly regulated to ensure efficient energy production.
In eukaryotes, these reactions take place in the mitochondria, while in prokaryotes, they occur in the cytoplasm. The enzymes involved in the Krebs cycle are located in the inner membrane or matrix space of the mitochondria. Most enzymes are present in the matrix, except for succinate dehydrogenase and aconitase.
The regulation of the Krebs cycle is influenced by various factors, including the presence of oxygen, levels of oxaloacetate or pyruvate, availability of necessary enzymes and coenzymes, as well as the levels of ATP and NADH.
Inhibition of the Krebs cycle can occur when any of these factors are disrupted, affecting the overall energy production in the cell.
Conclusion
The Krebs cycle is a crucial process in cellular respiration, occurring in different parts of cells depending on their type. Enzymes play a vital role in facilitating the chemical reactions of the cycle, leading to the production of high-energy molecules like NADH and FADH2, and the formation of ATP.
Understanding the location, enzymes, steps, and products of the Krebs cycle provides a deeper insight into cellular energy production.
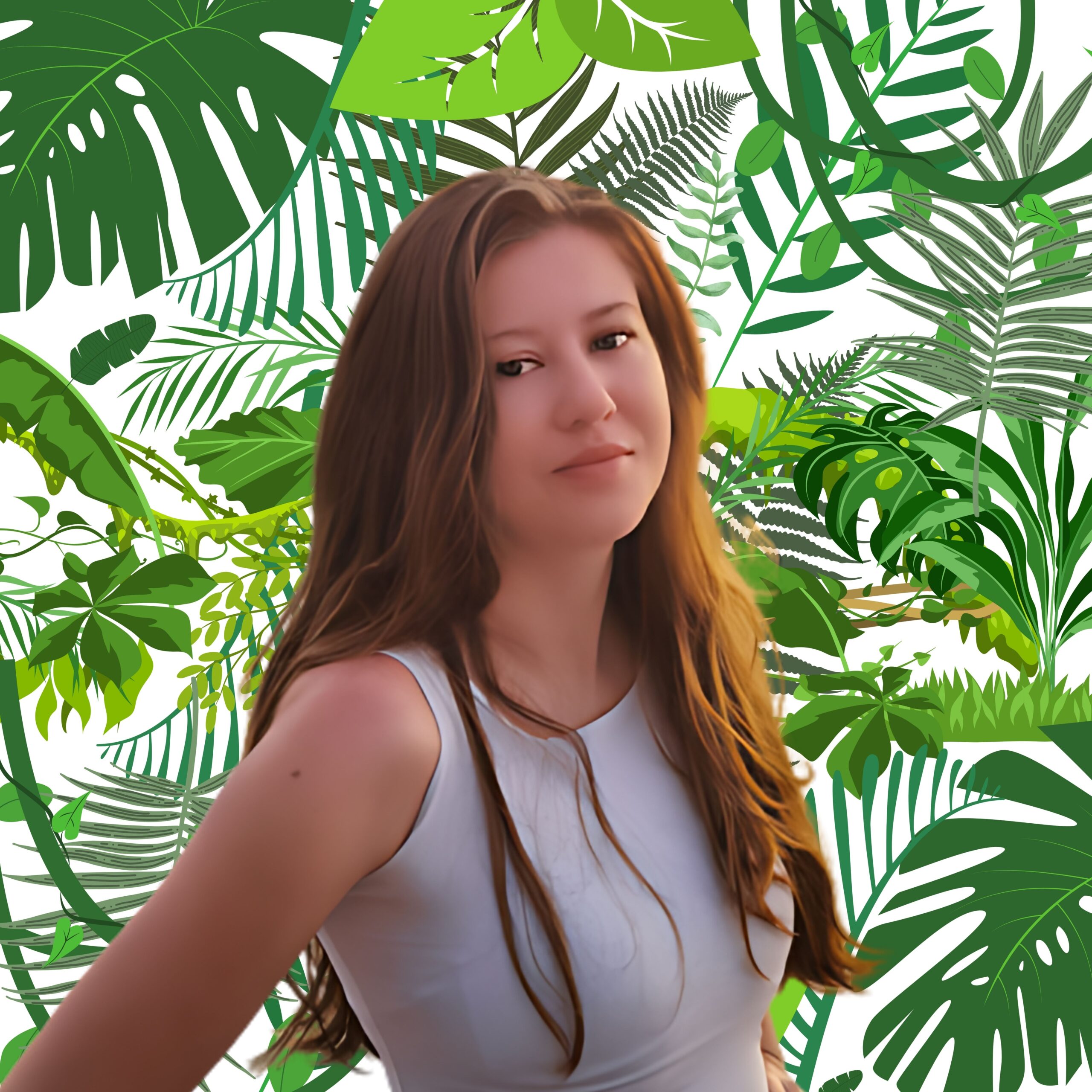
Erzsebet Frey (Eli Frey) is an ecologist and online entrepreneur with a Master of Science in Ecology from the University of Belgrade. Originally from Serbia, she has lived in Sri Lanka since 2017. Eli has worked internationally in countries like Oman, Brazil, Germany, and Sri Lanka. In 2018, she expanded into SEO and blogging, completing courses from UC Davis and Edinburgh. Eli has founded multiple websites focused on biology, ecology, environmental science, sustainable and simple living, and outdoor activities. She enjoys creating nature and simple living videos on YouTube and participates in speleology, diving, and hiking.