Photosynthetic pathways are the different methods plants use to convert sunlight, water, and CO2 into energy-rich sugars. There are three main types: C3, C4, and CAM. C3 is the most common, used by about 85% of plant species in moderate climates. C4 plants, like corn and sugarcane, thrive in hot, dry environments by concentrating CO2 around enzymes. CAM plants, such as cacti, adapt to arid conditions by fixing CO2 at night. These pathways have evolved to help plants survive in various environments and have significant implications for agriculture and climate change adaptation. Exploring these pathways can reveal fascinating insights into plant survival strategies.
The Basics of Photosynthesis
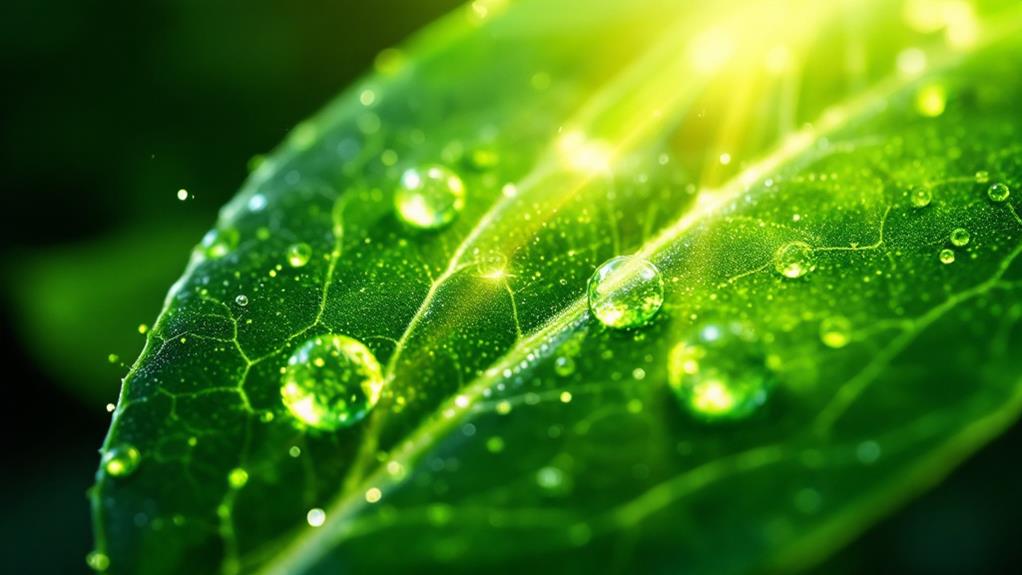
Photosynthesis is a fundamental process that powers life on Earth. It’s the remarkable ability of plants, algae, and some bacteria to convert light energy into chemical energy, producing the oxygen we breathe and the food we eat. You’ve likely heard about it in science class, but understanding its basics is essential to grasping more complex biological concepts.
At its core, photosynthesis involves two main stages: light-dependent reactions and light-independent reactions (also known as the Calvin cycle). In the light-dependent reactions, chlorophyll and other pigments in chloroplasts capture sunlight. This energy is used to split water molecules, releasing oxygen as a byproduct. The process also generates ATP and NADPH, which are energy-carrying molecules.
The light-independent reactions don’t directly require light but use the products from the light-dependent stage. Here, carbon dioxide from the air is converted into glucose using the energy stored in ATP and NADPH. This process is called carbon fixation.
The overall equation for photosynthesis is often simplified as:
6 CO₂ + 6 H₂O + light energy → C₆H₁₂O₆ + 6 O₂
You’ll notice that water and carbon dioxide are the reactants, while glucose and oxygen are the products. This elegant process not only provides energy for the plant but also forms the basis of most food chains on Earth. It’s a prime example of how organisms can harness external energy sources to drive their metabolic processes.
C3 Pathway: Nature’s Default
The workhorse of the plant world, the C3 pathway is the most common photosynthetic process found in nature. You’ll find it in about 85% of plant species, including trees, most crops, and grasses. This pathway gets its name from the first stable product of carbon fixation: a three-carbon compound called 3-phosphoglycerate.
In the C3 pathway, carbon dioxide enters the leaf through tiny pores called stomata. It’s then incorporated into organic compounds through a series of reactions known as the Calvin cycle. The key enzyme in this process is RuBisCO (Ribulose-1,5-bisphosphate carboxylase/oxygenase), which catalyzes the first major step of carbon fixation.
While efficient in moderate climates, the C3 pathway has its limitations. In hot, dry conditions, plants must close their stomata to conserve water, reducing CO2 intake. This can lead to photorespiration, a process where RuBisCO fixes oxygen instead of CO2, wasting energy and reducing photosynthetic efficiency.
Despite this drawback, C3 plants have adapted to thrive in diverse environments. They’re particularly successful in temperate regions and areas with consistent rainfall. Many important food crops, such as rice, wheat, and soybeans, use the C3 pathway.
Understanding the C3 pathway is vital for agricultural research and crop improvement. Scientists are working on enhancing RuBisCO efficiency and reducing photorespiration to boost crop yields and help plants adapt to changing climate conditions. As you explore deeper into photosynthetic pathways, you’ll see how nature has evolved alternative strategies to overcome the C3 pathway’s limitations.
C4 Pathway: Tropical Adaptation
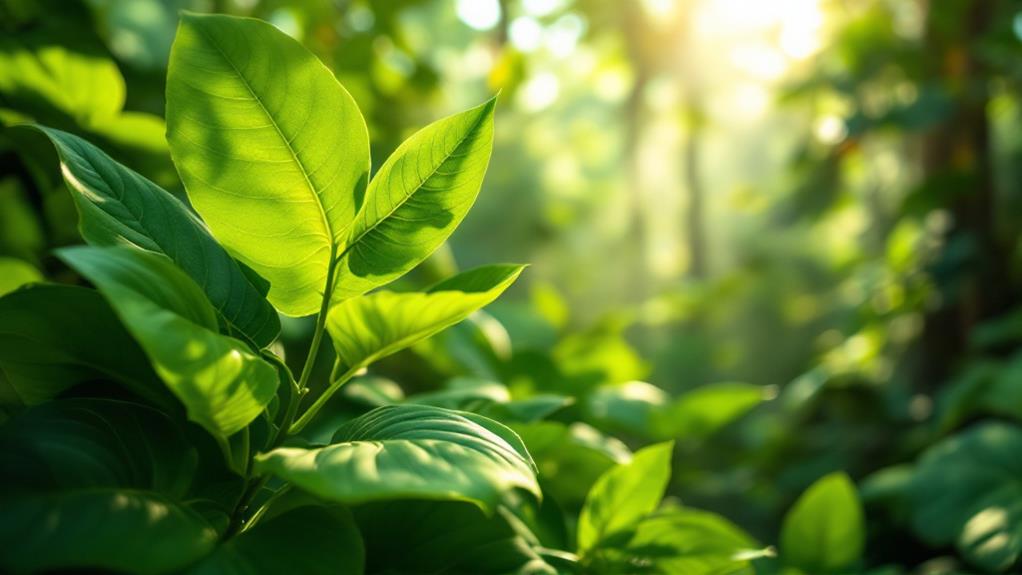
While C3 plants dominate temperate regions, nature has developed a more efficient process for hotter, drier climates. This adaptation is known as the C4 pathway, and it’s particularly prevalent in tropical and subtropical plants.
In C4 photosynthesis, you’ll find a unique anatomical structure called Kranz anatomy. This setup features two types of cells: mesophyll cells surrounding bundle sheath cells. The process begins in the mesophyll cells, where CO2 is fixed into a four-carbon compound by the enzyme PEP carboxylase. This initial step is more efficient at capturing CO2 than the C3 pathway, especially in high-temperature environments.
The four-carbon compound is then transported to the bundle sheath cells, where it’s broken down to release CO2. This creates a CO2-rich environment around RuBisCO, the enzyme responsible for carbon fixation in the Calvin cycle. By concentrating CO2, C4 plants minimize photorespiration, a process that wastes energy in C3 plants under hot, dry conditions.
You’ll find C4 photosynthesis in many important crops like corn, sugarcane, and sorghum. These plants can maintain high photosynthetic rates even when it’s hot and water is scarce. They’re also more efficient in their use of nitrogen and water compared to C3 plants.
However, the C4 pathway comes with a cost. It requires more energy to operate than the C3 pathway, making it less advantageous in cooler climates where photorespiration isn’t a significant issue. Despite this, the C4 pathway represents a remarkable evolutionary adaptation that’s allowed plants to thrive in challenging environments.
CAM Pathway: Desert Survival
Extreme environments demand extreme adaptations. In the harsh, arid conditions of deserts, plants have evolved a unique photosynthetic strategy called the CAM (Crassulacean Acid Metabolism) pathway. This ingenious adaptation allows plants to survive and thrive in environments where water is scarce and temperatures are extreme.
You’ll find that CAM plants have a distinct approach to carbon fixation. Unlike C3 and C4 plants, they open their stomata at night and close them during the day. This nocturnal activity minimizes water loss through transpiration, as nighttime temperatures are typically cooler and humidity is higher. During the night, CAM plants fix CO2 into organic acids, which are stored in vacuoles.
When daylight arrives, you’ll notice that CAM plants keep their stomata closed. They break down the stored organic acids, releasing CO2 internally. This CO2 is then used in the Calvin cycle for sugar production, all while the plant’s stomata remain shut, conserving precious water.
You’ll observe that CAM plants have thick, fleshy leaves or stems, which serve as efficient water storage organs. This adaptation allows them to endure prolonged periods of drought. Common examples of CAM plants you might encounter include cacti, pineapples, and various succulents.
While the CAM pathway is less efficient regarding overall carbon fixation compared to C3 and C4 pathways, it’s an essential survival mechanism in water-limited environments. You’ll find that some plants can switch between CAM and C3 pathways depending on environmental conditions, demonstrating the remarkable adaptability of desert flora.
Environmental Factors Influencing Pathways
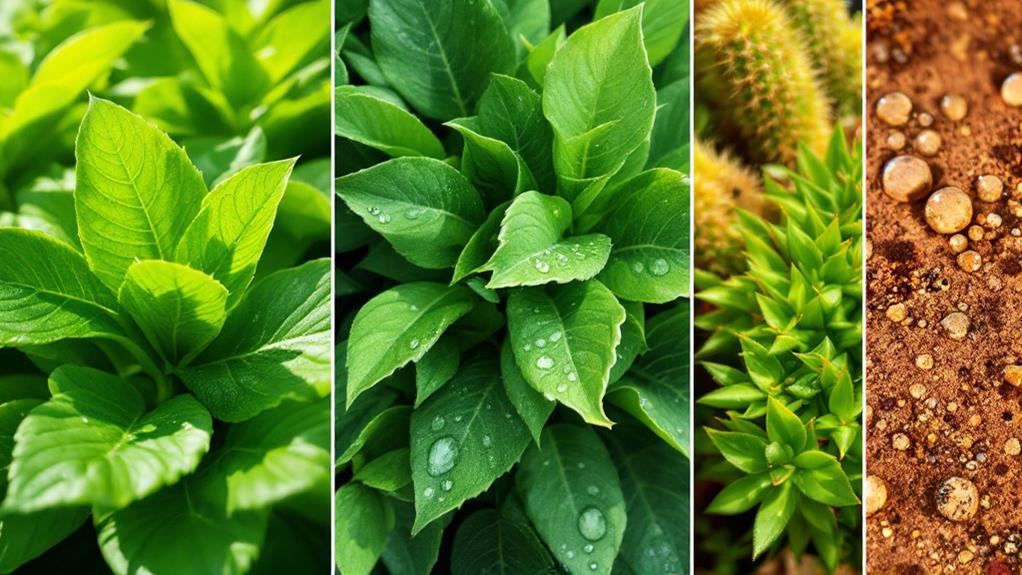
Environmental conditions play an essential role in determining which photosynthetic pathway a plant will utilize. You’ll find that temperature, light intensity, water availability, and CO2 concentration are the primary factors influencing these pathways.
In hot, dry environments with high light intensity, you’ll often see C4 and CAM plants thriving. These pathways help plants conserve water and minimize photorespiration, which becomes more prevalent in high temperatures. C4 plants, like corn and sugarcane, are particularly efficient in these conditions, as they can concentrate CO2 around RuBisCO, reducing oxygen competition.
When you’re in cooler, more temperate climates with moderate light intensity, you’ll encounter more C3 plants. This pathway is less energy-intensive and works well in environments where water isn’t as scarce. However, C3 plants struggle in hot, dry conditions due to increased photorespiration.
You’ll notice that CAM plants dominate in extremely arid environments. Their ability to open stomata at night and store CO2 as malic acid allows them to conserve water during the day. This adaptation is vital for survival in desert ecosystems.
CO2 concentration also affects pathway selection. In environments with low CO2 levels, C4 and CAM plants have an advantage over C3 plants. However, as atmospheric CO2 increases due to climate change, you might see C3 plants becoming more competitive in some areas.
Understanding these environmental influences helps you predict plant distributions and adapt agricultural practices to changing climates. It’s a vital aspect of plant ecology and crop science that you’ll need to take into account when studying or working with plants in various ecosystems.
Evolution of Photosynthetic Pathways
When you investigate the evolution of photosynthetic pathways, you’re exploring a fascinating journey of plant adaptation. The story begins with the C3 pathway, which evolved around 3 billion years ago in cyanobacteria and remains the most common form of photosynthesis today. This ancient pathway is found in most plants and is well-suited for cooler, wetter environments.
As Earth’s climate changed and CO2 levels fluctuated, some plants developed more efficient ways to capture carbon. About 30-40 million years ago, the C4 pathway emerged independently in multiple plant lineages. This adaptation allows plants to concentrate CO2 around RuBisCO, reducing photorespiration and improving efficiency in hot, dry conditions.
You’ll find that the CAM pathway evolved even more recently, roughly 16 million years ago. It’s an adaptation that enables plants to conserve water by opening their stomata at night to collect CO2. This pathway is particularly beneficial in arid environments.
It’s important to note that these pathways continue to evolve. You can observe ongoing adaptations in plants like Flaveria, which exhibit intermediate C3-C4 states. This suggests that evolution towards more efficient carbon fixation is still occurring.
Understanding the evolution of photosynthetic pathways isn’t just about plant history. It’s essential for predicting how plants might adapt to future climate changes and for developing more resilient crops. As you explore deeper into this topic, you’ll uncover the intricate ways plants have responded to environmental pressures throughout Earth’s history.
Agricultural Implications
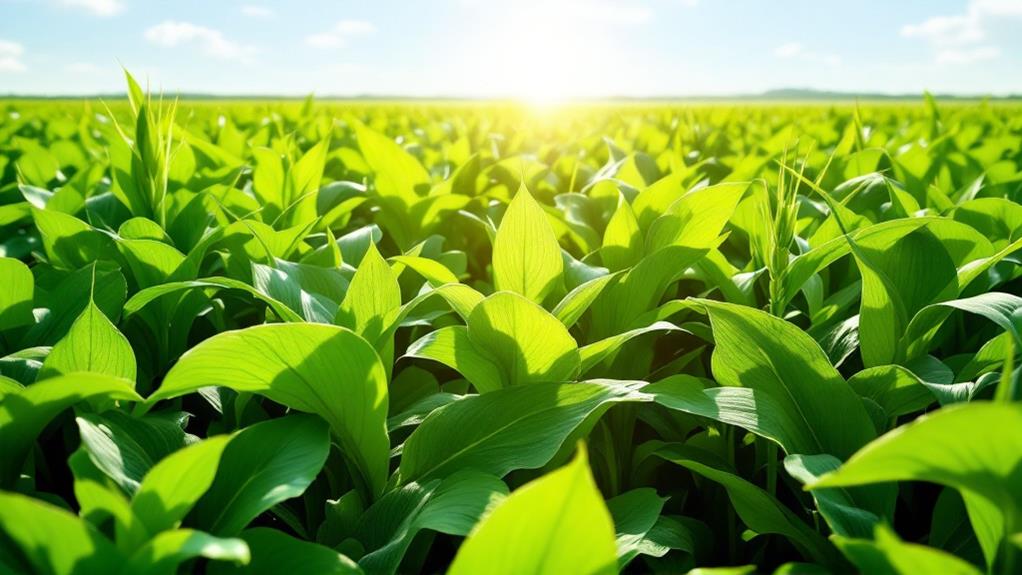
The evolution of photosynthetic pathways has profound implications for agriculture. Understanding these pathways can help you improve crop yields, select suitable plants for specific environments, and develop more efficient farming practices.
C3 plants, which include major crops like wheat, rice, and soybeans, are well-suited for temperate climates with moderate light intensity and temperature. They’re efficient in cooler, wetter conditions but struggle in hot, dry environments. You’ll find C3 crops dominating agriculture in regions with ample rainfall and moderate temperatures.
C4 plants, such as corn, sugarcane, and sorghum, thrive in warmer, drier climates. They’re more efficient at using water and nitrogen, making them ideal for regions with high light intensity and temperature. You’ll see C4 crops increasingly important in areas affected by climate change, as they’re better equipped to handle drought and heat stress.
CAM plants, like pineapples and agave, are adapted to arid environments. While they’re not as widely cultivated as C3 or C4 crops, they’re valuable in water-scarce regions. You can use CAM plants for sustainable agriculture in areas prone to drought or desertification.
Future Research and Applications
Scientists continue to explore new frontiers in photosynthesis research, aiming to reveal its full potential for addressing global challenges. You’ll find that current research focuses on enhancing crop productivity, developing sustainable energy sources, and mitigating climate change effects.
One exciting area of study is the engineering of C4 photosynthesis into C3 plants. This could greatly boost yields in staple crops like rice and wheat. You’ll see researchers working on introducing CO2-concentrating mechanisms and optimizing Rubisco enzyme efficiency to achieve this goal.
Another promising field is artificial photosynthesis. Scientists are developing synthetic systems that mimic natural photosynthetic processes to produce clean fuels and valuable chemicals. You can expect breakthroughs in water-splitting catalysts and CO2 reduction technologies in the coming years.
Algal biofuels represent a sustainable alternative to fossil fuels. Researchers are focusing on improving algal strains, cultivation methods, and harvesting techniques to make this technology economically viable. You’ll likely witness notable advancements in photobioreactor designs and genetic engineering of algae for enhanced oil production.
The study of cyanobacteria for carbon capture and bioremediation is gaining traction. You’ll find scientists exploring ways to harness these microorganisms’ photosynthetic capabilities to remove pollutants from water and air while sequestering atmospheric CO2.
As you look to the future, expect to see photosynthesis research playing a vital role in developing climate-resilient crops, creating sustainable materials, and innovating in fields like medicine and nanotechnology.
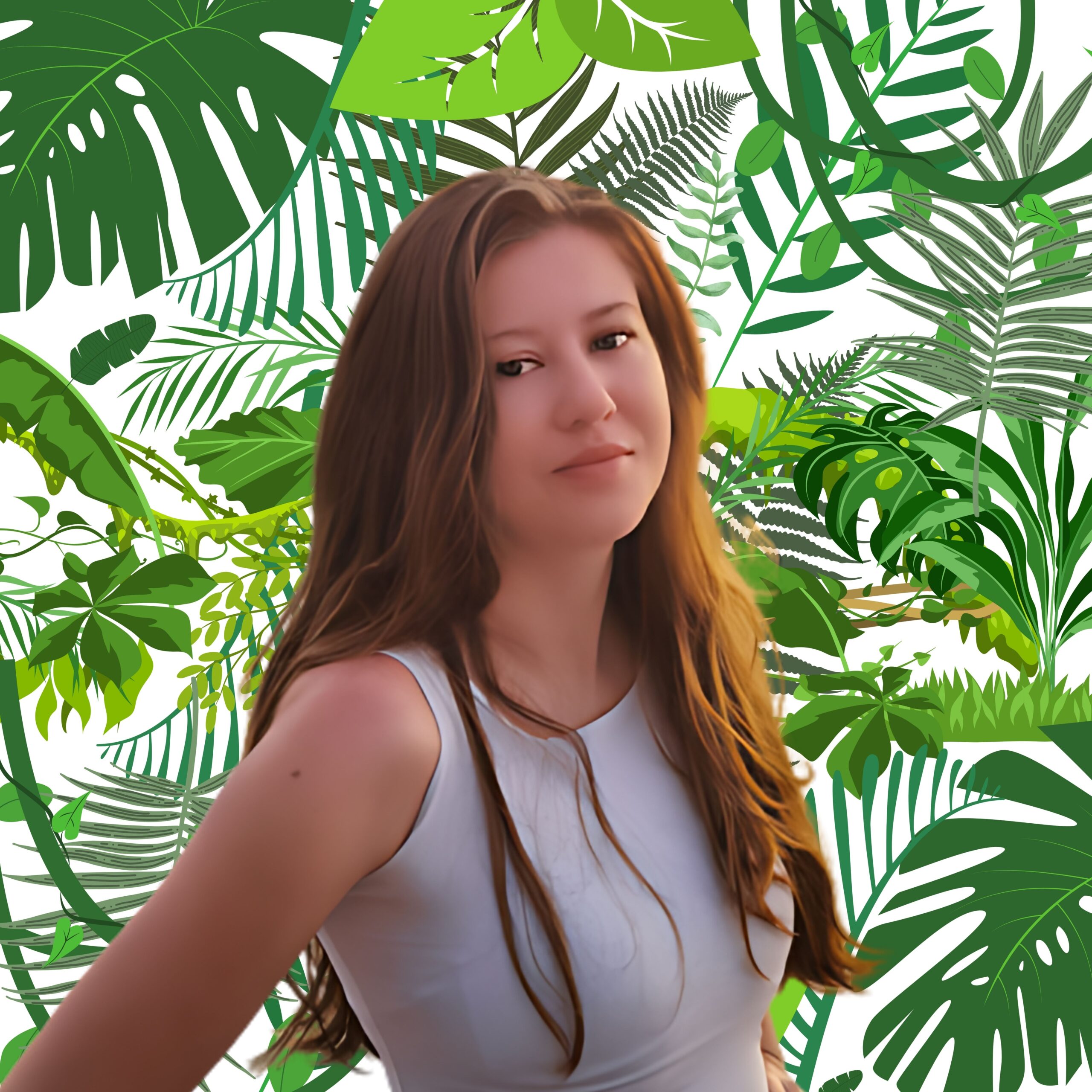
Erzsebet Frey (Eli Frey) is an ecologist and online entrepreneur with a Master of Science in Ecology from the University of Belgrade. Originally from Serbia, she has lived in Sri Lanka since 2017. Eli has worked internationally in countries like Oman, Brazil, Germany, and Sri Lanka. In 2018, she expanded into SEO and blogging, completing courses from UC Davis and Edinburgh. Eli has founded multiple websites focused on biology, ecology, environmental science, sustainable and simple living, and outdoor activities. She enjoys creating nature and simple living videos on YouTube and participates in speleology, diving, and hiking.