Microbiology is the study of microscopic organisms, including bacteria, viruses, fungi, and protozoa. You'll discover how these tiny life forms impact our world in countless ways, from the beneficial roles they play in ecosystems to their potential to cause diseases. Understanding microbiology is essential for fields like medicine, agriculture, and biotechnology, where knowledge of microorganisms is applied to develop treatments, improve crop yields, and create innovative products. You'll also learn about methods to control microbial growth, such as sterilization, disinfection, and the use of antimicrobial agents, as well as the importance of vaccination in preventing infectious diseases. Keep exploring to uncover the enthralling world of microbes.
Microorganisms
Microorganisms are everywhere, even though you can't see them with the naked eye. They're in the air you breathe, the food you eat, and on every surface you touch. These tiny living things are classified into different groups based on their characteristics, such as bacteria, viruses, fungi, protozoa, and algae.
Bacteria are single-celled organisms that come in various shapes, like rods, spheres, or spirals. Some are helpful, like those in your gut that aid digestion, while others can cause diseases. Viruses are even smaller than bacteria and need a host cell to replicate. They're responsible for illnesses like the flu and COVID-19.
Fungi include yeasts, molds, and mushrooms. Some are used in food production, like baker's yeast for bread, while others can cause infections. Protozoa are single-celled organisms that live in water or damp environments. Some are harmless, but others can cause diseases like malaria.
Algae are plant-like organisms that can be single-celled or multicellular. They're significant for the environment, producing oxygen and serving as a food source for other organisms.
Understanding microorganisms is essential for fields like medicine, agriculture, and biotechnology. Researchers study them to develop new treatments, improve crop yields, and create innovative products. So, the next time you can't see something, remember that there's a whole world of microorganisms around you, playing important roles in our lives and ecosystems.
Bacteria
Bacteria are the most abundant microorganisms on Earth, found in nearly every environment imaginable. They're single-celled prokaryotes that lack a nucleus and other membrane-bound organelles. Despite their small size, bacteria play vital roles in ecosystems, from decomposing organic matter to fixing nitrogen in the soil.
You'll find bacteria in various shapes, including spherical (cocci), rod-shaped (bacilli), and spiral (spirilla). Some bacteria form colonies, while others live independently. They reproduce through binary fission, quickly multiplying under favorable conditions.
Many bacteria are beneficial to humans, such as those in your gut that aid digestion and produce vitamins. However, some bacteria cause diseases like strep throat, pneumonia, and food poisoning. Pathogenic bacteria can invade host cells, produce toxins, or trigger immune responses that lead to illness.
Bacteria are classified based on their cell wall structure, which is determined by Gram staining. Gram-positive bacteria have a thick cell wall, while Gram-negative bacteria have a thinner cell wall and an outer membrane. This distinction is important for identifying bacteria and selecting appropriate antibiotics.
Antibiotics are substances that kill or inhibit the growth of bacteria. However, overuse and misuse of antibiotics have led to the emergence of antibiotic-resistant bacteria, posing significant public health challenges.
Understanding the diversity, structure, and function of bacteria is essential for fields like medicine, biotechnology, and environmental science. By studying these microscopic organisms, we can develop new ways to combat diseases, harness their metabolic capabilities, and maintain ecological balance.
Viruses
Unlike bacteria, viruses are not considered living organisms because they lack the ability to reproduce independently. They're incredibly small, ranging from 20 to 400 nanometers in diameter, and can only be seen with an electron microscope. Viruses consist of genetic material (DNA or RNA) enclosed in a protein coat called a capsid, and some have an additional outer envelope.
To replicate, viruses must infect a host cell and hijack its cellular machinery. They attach to specific receptors on the host cell's surface and inject their genetic material inside. The viral genes then direct the host cell to produce viral components, which assemble into new viruses. These viruses burst out of the cell, destroying it in the process, and go on to infect other cells.
Viruses can infect all types of life forms, from animals and plants to bacteria and archaea. They cause numerous diseases in humans, such as the common cold, influenza, measles, and COVID-19. While antibiotics are ineffective against viruses, vaccines can prevent viral infections, and antiviral medications can help manage symptoms and reduce the duration of illness.
Researchers also use viruses as tools in molecular biology and gene therapy. By modifying viruses to carry specific genes, scientists can introduce desired genetic material into cells for research or therapeutic purposes. Understanding the structure, replication, and behavior of viruses is essential for developing effective strategies to prevent and treat viral diseases, as well as harnessing their potential in scientific applications.
Fungi
Fungi are eukaryotic organisms that play vital roles in various ecosystems. They're distinct from plants and animals, belonging to their own kingdom called Fungi. Fungi can be both unicellular and multicellular, and they obtain nutrients by absorbing organic compounds from their surroundings. This process is called absorption, and it's facilitated by the presence of a cell wall made of chitin.
Fungi reproduce through spores, which can be asexual or sexual. Asexual reproduction occurs through fragmentation, budding, or the production of spores, while sexual reproduction involves the fusion of two compatible haploid cells to form a diploid zygote. The zygote then undergoes meiosis to produce haploid spores, which can germinate and grow into new individuals.
Fungi are classified into four main phyla: Ascomycota, Basidiomycota, Chytridiomycota, and Zygomycota. Ascomycota includes yeasts, molds, and mushrooms that produce spores in sac-like structures called asci. Basidiomycota comprises mushrooms, puffballs, and rust fungi, which produce spores on club-shaped cells called basidia. Chytridiomycota are mainly aquatic fungi that have flagellated spores, while Zygomycota are mainly terrestrial and produce spores in specialized structures called sporangia.
Fungi play important roles in ecosystems as decomposers, breaking down dead organic matter and recycling nutrients back into the environment. They also form symbiotic relationships with other organisms, such as lichens and mycorrhizae. Some fungi are pathogenic and can cause diseases in plants, animals, and humans, while others are used in the production of food, beverages, and pharmaceuticals.
Protozoa
Tiny, single-celled organisms known as protozoa are eukaryotic microbes that belong to the kingdom Protista. They're found in various habitats, including soil, freshwater, and marine environments. Some protozoa are even parasitic, living inside the bodies of other organisms. Unlike bacteria, protozoa have a membrane-bound nucleus and other organelles, such as mitochondria and the endoplasmic reticulum.
Protozoa exhibit diverse methods of locomotion, depending on the species. Flagellates move using whip-like structures called flagella, while ciliates are covered in tiny hair-like projections called cilia. Amoebae, on the other hand, move by extending their cytoplasm to form temporary projections called pseudopodia.
Nutrition in protozoa also varies. Some species are photosynthetic, like algae, while others are heterotrophic, obtaining nutrients by ingesting bacteria, algae, or other protozoa. Parasitic protozoa, such as Plasmodium (which causes malaria), absorb nutrients from their hosts.
Protozoa play vital roles in ecosystems, acting as predators and prey in microbial food webs. They also contribute to nutrient cycling by breaking down organic matter. However, some protozoa can cause diseases in humans and animals. For example, Giardia lamblia causes giardiasis, a diarrheal illness, while Trypanosoma species cause sleeping sickness and Chagas disease.
Understanding the biology and ecology of protozoa is essential for managing their roles in the environment and in human and animal health. Researchers continue to study these fascinating microorganisms to unravel their complexities and develop strategies for controlling the spread of protozoan diseases.
Archaea
Archaea represent another domain of single-celled microorganisms that are prokaryotic, like bacteria, but they're genetically and biochemically distinct. They lack a nucleus and membrane-bound organelles, and their cell walls don't contain peptidoglycan, which is found in bacterial cell walls. Instead, archaeal cell walls are made of pseudopeptidoglycan or protein.
You'll find archaea in various environments, including extreme habitats like hot springs, salt lakes, and deep-sea hydrothermal vents. Some archaea are methanogens, producing methane as a byproduct of their metabolism, while others are halophiles, thriving in high-salt environments, or thermophiles, living in extremely hot conditions.
Archaea play essential roles in the carbon and nitrogen cycles. Methanogenic archaea are responsible for the production of methane in anaerobic environments, such as wetlands and the digestive tracts of ruminants. This methane contributes to the greenhouse effect and global warming.
Despite their similarities to bacteria, archaea have unique features in their DNA replication, transcription, and translation processes. They also have distinct lipid compositions in their cell membranes, which help them adapt to extreme environments.
Research on archaea has led to advancements in biotechnology.
Microbial Taxonomy
Microbial taxonomy is the science of naming and classifying microorganisms into groups based on shared characteristics. It's a critical field in microbiology, as it helps researchers understand the relationships between different microbes and their roles in various ecosystems. The classification system is hierarchical, with the main ranks being domain, kingdom, phylum, class, order, family, genus, and species. Each rank represents a more specific grouping of organisms with increasingly similar traits.
To classify microbes, taxonomists consider morphological, biochemical, and genetic characteristics. Morphological features include cell shape, size, and presence of structures like flagella or capsules. Biochemical traits involve metabolic capabilities, such as the ability to ferment certain sugars or produce specific enzymes. Genetic analysis, particularly DNA sequencing, has revolutionized microbial taxonomy by providing a more accurate and reliable means of determining evolutionary relationships.
The two main domains of microorganisms are Bacteria and Archaea. Within these domains, you'll find a wide variety of kingdoms, phyla, and lower ranks. Some notable examples include the Proteobacteria phylum, which contains many common bacterial pathogens, and the Fungi kingdom, which includes yeasts and molds. As you explore deeper into microbiology, you'll encounter numerous fascinating examples of microbial diversity, all organized according to the principles of taxonomy.
Bacterial Cell Structure
Comprised of several distinct components, the bacterial cell structure is a fascinating study in microbiology. You'll find that the bacterial cell is surrounded by a cell wall, which provides protection and maintains the cell's shape. Beneath the cell wall lies the cell membrane, a selective barrier controlling the movement of substances in and out of the cell. The cytoplasm, a jelly-like substance, contains ribosomes, enzymes, and other cellular components indispensable for bacterial growth and metabolism.
You'll also discover that many bacteria possess additional structures, such as flagella for motility, pili for attachment, and a capsule for extra protection. Some bacteria form endospores, allowing them to survive harsh conditions. The bacterial genome, consisting of a single circular DNA molecule, is located in the cytoplasm, unlike eukaryotic cells, which have a membrane-bound nucleus.
Understanding the bacterial cell structure is vital for comprehending how bacteria function, interact with their environment, and respond to antibiotics. By studying the unique features of bacterial cells, you'll gain insights into their diverse roles in ecosystems, their potential as beneficial microbes, and their ability to cause diseases. This knowledge is essential for developing strategies to control harmful bacteria and harness the power of beneficial ones in various fields, from medicine to biotechnology.
Viral Structure
They may be small, but viruses pack a punch when it comes to their structure and function. You might think of them as tiny packets of genetic material, but they're much more complex than that. A typical virus consists of a core of DNA or RNA, surrounded by a protein coat called a capsid. Some viruses also have an outer envelope made of lipids and proteins.
The capsid protects the viral genome and helps the virus attach to and enter host cells. It's made up of repeating protein subunits that self-assemble into a symmetrical structure, such as an icosahedron or a helix. The capsid's shape and size vary among different viruses, and they play a significant role in determining the virus's host range and infectivity.
The viral genome contains the genetic instructions for replicating the virus and making new viral proteins. It can be single-stranded or double-stranded, and it can be DNA or RNA. Some viruses have segmented genomes, while others have a single, continuous molecule.
The envelope, when present, is derived from the host cell's membrane during the viral budding process. It contains viral glycoproteins that help the virus bind to and enter new host cells. Enveloped viruses are generally more susceptible to environmental factors, such as heat and drying, compared to non-enveloped viruses.
Understanding viral structure is vital for developing antiviral drugs and vaccines. By targeting specific components of the viral structure, scientists can disrupt the viral life cycle and prevent infection.
Fungal Cell Structure
Fungi are eukaryotic organisms with a unique cell structure that sets them apart from other microbes. They have a thick cell wall composed of chitin, a complex polysaccharide that provides structural support and protection. Unlike plant cells, fungal cells lack chloroplasts, as they don't perform photosynthesis. Instead, they obtain nutrients through absorption.
The plasma membrane of fungal cells is similar to that of other eukaryotic cells, but it contains ergosterol instead of cholesterol. This difference is exploited by antifungal drugs that target ergosterol synthesis. Inside the cell, you'll find organelles such as the nucleus, endoplasmic reticulum, Golgi apparatus, and mitochondria.
Fungal cells also have specialized structures called hyphae, which are long, branching filaments that form the mycelium, the vegetative part of a fungus. Hyphae can be septate, with cross-walls dividing the cells, or aseptate, lacking these divisions. Some fungi have specialized hyphae called haustoria, which penetrate host cells to absorb nutrients.
Fungal cells reproduce through the formation of spores, which can be asexual or sexual. Asexual spores, such as conidia, are produced by mitosis, while sexual spores, like ascospores or basidiospores, result from meiosis. Understanding the unique features of fungal cell structure is essential for developing effective strategies to control fungal growth and combat fungal infections.
Protozoan Cell Structure
Protozoans, the tiny single-celled eukaryotes, boast a fascinating array of cellular structures that enable their diverse lifestyles. Their cell membrane, a phospholipid bilayer, acts as a selective barrier, regulating the movement of molecules in and out of the cell. Beneath the membrane lies the cytoplasm, a gel-like substance that houses the organelles vital for the protozoan's survival.
One of the most prominent organelles is the nucleus, which contains the protozoan's genetic material. The nucleus is surrounded by a double membrane, the nuclear envelope, which has pores that allow for the selective transport of molecules between the nucleus and the cytoplasm.
Protozoans also possess mitochondria, the powerhouses of the cell, which generate ATP through cellular respiration. The endoplasmic reticulum, a network of membrane-bound channels, plays a significant role in protein and lipid synthesis, while the Golgi apparatus modifies, packages, and distributes these molecules.
Unique to protozoans are specialized structures like the pellicle, a flexible outer covering that provides support and protection, and the contractile vacuole, which helps maintain water balance in freshwater species. Some protozoans also have specialized feeding structures, such as the oral groove in Paramecium, which directs food particles into the cell.
The presence of these organelles and specialized structures allows protozoans to carry out complex functions, such as movement, feeding, and reproduction, despite their small size. Understanding the intricacies of protozoan cell structure is key to appreciating the remarkable adaptations of these diverse microorganisms.
Microbial Metabolism
At the heart of every living organism lies a complex network of chemical reactions, a symphony of life-sustaining processes known as metabolism. As you explore the world of microbiology, you'll discover that microbial metabolism is a fascinating and diverse field. Microbes, despite their tiny size, possess an incredible array of metabolic pathways that allow them to thrive in various environments.
You'll learn about the two main types of microbial metabolism: catabolism and anabolism. Catabolism involves the breakdown of complex molecules to release energy and simple building blocks, while anabolism uses these building blocks and energy to synthesize new cellular components. You'll investigate how microbes harness energy from different sources, such as light, organic compounds, and inorganic substances, through processes like photosynthesis, fermentation, and respiration.
You'll also discover the significance of enzymes in microbial metabolism, as these biological catalysts speed up chemical reactions and enable microbes to perform complex transformations efficiently. As you progress in your studies, you'll encounter the intricate metabolic pathways that allow microbes to adapt to diverse environments, from the depths of the ocean to the human gut. Understanding microbial metabolism is essential for harnessing the power of microbes in biotechnology, medicine, and environmental science. So, get ready to unravel the mysteries of microbial metabolism and appreciate the incredible metabolic versatility of these tiny yet mighty organisms.
Bacterial Reproduction
When you plunge into the world of microbiology, you'll encounter the fascinating process of bacterial reproduction. Most bacteria reproduce asexually through binary fission, where a single cell divides into two genetically identical daughter cells. This process begins with DNA replication, followed by the segregation of the duplicated chromosomes to opposite ends of the cell. A septum forms, separating the cell into two compartments, and the cell wall constricts, eventually splitting the original cell into two.
Some bacteria also reproduce through a process called conjugation, which involves the transfer of genetic material between two cells via a specialized structure called a pilus. The donor cell transfers a portion of its DNA, called a plasmid, to the recipient cell, resulting in genetic recombination.
Bacterial reproduction is influenced by various environmental factors, such as nutrient availability, temperature, and pH. Under favorable conditions, bacteria can reproduce rapidly, with some species capable of doubling their population every 20 minutes. This exponential growth allows bacteria to quickly adapt to their environment and colonize new niches.
Understanding bacterial reproduction is essential for various applications, from the development of antibiotics to the production of biotechnology products. By studying the mechanisms and regulation of bacterial reproduction, microbiologists can develop strategies to control bacterial growth, combat infectious diseases, and harness the power of these microorganisms for the benefit of society.
Viral Replication
Unlike bacteria, viruses are non-living entities that rely on host cells for replication. Viral replication is a complex process that involves multiple steps, each critical for the successful production of new virus particles. First, the virus attaches to a specific receptor on the host cell surface and injects its genetic material (DNA or RNA) into the cell. Once inside, the viral genome hijacks the host cell's machinery, forcing it to produce viral components such as proteins and nucleic acids.
Next, these components assemble into new virus particles, which accumulate within the host cell. The assembly process varies depending on the type of virus, but generally involves the packaging of the viral genome into a protective protein shell called a capsid. Some viruses also acquire an envelope derived from the host cell membrane.
Fungal Reproduction
Fungi, the fascinating eukaryotic organisms, have evolved diverse strategies for reproduction. You'll find that fungi can reproduce both sexually and asexually, depending on the species and environmental conditions. Asexual reproduction occurs through fragmentation, budding, or the formation of spores. In fragmentation, a piece of the fungal mycelium breaks off and grows into a new individual. Budding involves the formation of a small outgrowth that separates from the parent cell. Spores, which are reproductive structures, can be produced in large numbers and dispersed by wind or water.
Sexual reproduction in fungi involves the fusion of two compatible haploid cells to form a diploid zygote. This process is called plasmogamy. The zygote then undergoes karyogamy, where the two nuclei fuse to form a diploid nucleus. Meiosis follows, producing haploid spores that can germinate into new individuals. Some fungi have specialized structures for sexual reproduction, such as mushrooms, which are the fruiting bodies that produce spores.
The type of reproduction employed by a fungal species depends on various factors, including nutrient availability, temperature, and the presence of a compatible mate. Some fungi can switch between sexual and asexual reproduction, allowing them to adapt to changing conditions. Understanding fungal reproduction is essential for managing fungal infections, controlling fungal growth in food production, and harnessing their potential in biotechnology applications.
Protozoan Reproduction
Protozoa, single-celled eukaryotic organisms, also have fascinating reproductive strategies. You'll find that most protozoa reproduce asexually through binary fission, where the parent cell divides into two identical daughter cells. This process involves DNA replication, followed by the separation of the duplicated genetic material and organelles into the newly formed cells. Some protozoa, like Paramecium, also undergo a type of sexual reproduction called conjugation. During conjugation, two cells exchange genetic material through a temporary cytoplasmic bridge, resulting in genetic recombination and increased genetic diversity.
Certain protozoa, such as Plasmodium (the causative agent of malaria), have complex life cycles that involve both asexual and sexual reproduction in different hosts. In the mosquito vector, Plasmodium undergoes sexual reproduction, forming gametes that fuse to create a zygote. The zygote then develops into an ookinete, which penetrates the mosquito's gut wall and forms an oocyst. Inside the oocyst, the parasite undergoes multiple rounds of asexual reproduction, producing numerous sporozoites that migrate to the mosquito's salivary glands, ready to infect a new host during the next blood meal. Understanding the reproductive strategies of protozoa is vital for developing effective control measures against parasitic species and for appreciating the diversity of life at the microscopic level.
Microbial Genetics
Microbial genetics is a fascinating field that explores the genetic material and mechanisms of inheritance in microorganisms. You'll discover that bacteria and archaea typically have a single circular chromosome, while eukaryotic microbes like fungi and protists have multiple linear chromosomes. Plasmids, which are small, circular DNA molecules, are also common in bacteria and yeasts. They often carry genes for antibiotic resistance or other selective advantages.
Microbial genomes are incredibly diverse, with sizes ranging from a few hundred thousand to several million base pairs. They evolve rapidly through mutations, recombination, and horizontal gene transfer. This allows microbes to adapt quickly to changing environments and acquire new capabilities.
Genetic tools like PCR, sequencing, and cloning have revolutionized the study of microbial genetics. They've enabled the identification of genes responsible for specific traits, the creation of genetically modified organisms, and the development of new biotechnology applications.
Understanding microbial genetics is essential for fields like medicine, agriculture, and environmental science. It helps us combat antibiotic resistance, engineer microbes for bioremediation, and develop new vaccines and therapies. As you explore deeper into this subject, you'll appreciate the incredible power and potential of these tiny genetic powerhouses.
Bacterial Transformation
Have you ever wondered how bacteria can gain new abilities, like antibiotic resistance? It's through a process called bacterial transformation. Transformation occurs when a bacterium takes up foreign DNA from its environment and incorporates it into its own genome. This can happen naturally, but scientists have also developed methods to artificially induce transformation in the lab.
For transformation to occur, the recipient bacterium must be in a state of competence, which means it's able to take up DNA from its surroundings. Some bacteria are naturally competent, while others require specific treatments to become competent. Once the foreign DNA enters the cell, it can be integrated into the bacterium's genome through recombination.
Transformation has many applications in molecular biology and biotechnology. It's used to introduce recombinant DNA into bacteria for gene cloning, protein expression, and genetic engineering. By transforming bacteria with plasmids containing genes of interest, researchers can study gene function, produce large quantities of proteins, and create genetically modified organisms.
Bacterial transformation is a powerful tool that has revolutionized our understanding of genetics and opened up new possibilities for medicine and industry. It's a key concept in microbiology that illustrates the incredible adaptability and versatility of bacteria. So the next time you hear about a new antibiotic-resistant strain of bacteria, remember that transformation may have played a role in its emergence.
Viral Transduction
Viruses have a sneaky way of transferring genetic material between bacteria, and it's called transduction. In this process, a virus known as a bacteriophage infects a bacterial cell and incorporates a piece of the host's DNA into its own viral genome. When the virus replicates and bursts out of the cell, it carries this bacterial DNA with it. If the virus then infects another bacterial cell, it can inject the previously acquired DNA into the new host, effectively transferring genetic material from one bacterium to another.
There are two main types of transduction: generalized and specialized. In generalized transduction, any piece of bacterial DNA can be packaged into the virus and transferred to another cell. Specialized transduction, on the other hand, occurs when the virus mistakenly packages a specific piece of DNA located next to its own integration site in the bacterial genome.
Transduction plays a significant role in bacterial evolution, allowing them to acquire new genes and traits, such as antibiotic resistance or virulence factors. It's also a valuable tool in genetic engineering, as scientists can use viruses to introduce desired genes into bacterial cells. Understanding the mechanisms of transduction is essential for developing strategies to combat the spread of harmful traits among bacterial populations and for harnessing its potential in biotechnology applications.
Microbial Ecology
Beneath the surface of every ecosystem lies a hidden world teeming with microscopic life. Microbial ecology is the study of these microorganisms, their interactions with each other, and their environment. It's a fascinating field that explores the diverse roles microbes play in shaping our world.
Microbes are found in every habitat on Earth, from the depths of the ocean to the highest mountain peaks. They form complex communities, engaging in intricate relationships that drive nutrient cycling, decomposition, and other essential ecological processes. Some microbes form symbiotic relationships with plants and animals, while others compete for resources or prey on each other.
Microbial ecologists use a variety of techniques to study these communities, including DNA sequencing, microscopy, and chemical analysis. They aim to understand how microbial diversity and function are influenced by environmental factors like temperature, pH, and nutrient availability. By unraveling these complex interactions, we can better appreciate the crucial role microbes play in maintaining the health and balance of ecosystems worldwide.
As you explore deeper into microbial ecology, you'll discover a world of incredible adaptations and survival strategies. From extremophiles thriving in harsh conditions to bacteria communicating through chemical signals, the microbial world is full of wonders. By studying these tiny organisms, we gain insights into the very foundations of life on Earth and the potential for microbes to help solve some of our most pressing environmental challenges.
Human Microbiome
You're not just a single organism; you're a walking ecosystem. The human microbiome consists of trillions of microbes that inhabit your body, including bacteria, fungi, and viruses. These microbes outnumber your own cells by a ratio of about 10 to 1. The majority of these microbes reside in your gut, but they also inhabit your skin, mouth, and other parts of your body.
Your microbiome plays a vital role in maintaining your health. It helps digest your food, synthesize essential vitamins and nutrients, and regulate your immune system. Imbalances in your microbiome have been linked to various health issues, such as obesity, diabetes, and inflammatory bowel disease.
The composition of your microbiome is influenced by factors like your diet, lifestyle, and antibiotic use. Eating a diverse, fiber-rich diet and avoiding unnecessary antibiotics can help maintain a healthy microbiome. Probiotics and prebiotics may also be beneficial in promoting the growth of beneficial microbes.
Research into the human microbiome is rapidly expanding, and scientists are exploring ways to manipulate the microbiome to prevent and treat diseases. As you learn more about your microbiome, you'll appreciate the complex symbiotic relationship between you and your microbial inhabitants, and how this relationship shapes your overall health and well-being.
Soil Microbiology
The hidden world of soil is teeming with microscopic life. You'll find an incredibly diverse community of microorganisms, including bacteria, fungi, protozoa, and algae, all playing essential roles in the soil ecosystem. These microbes are significant for nutrient cycling, decomposition of organic matter, and maintaining soil health.
Soil bacteria are the most abundant microorganisms, with a single gram of soil containing billions of bacterial cells. They're involved in nitrogen fixation, converting atmospheric nitrogen into forms usable by plants. Fungi, such as mycorrhizae, form symbiotic relationships with plant roots, enhancing nutrient and water uptake. They also break down complex organic compounds, releasing nutrients back into the soil.
Protozoa and algae are less numerous but still important. Protozoa feed on bacteria and fungi, regulating their populations and releasing nutrients. Algae contribute to soil fertility through photosynthesis and nitrogen fixation.
Soil microbiology has implications for agriculture, environmental management, and bioremediation. Understanding the interactions between microbes and their environment helps develop sustainable farming practices, improve soil quality, and address issues like soil contamination. Techniques such as soil inoculation with beneficial microbes can enhance plant growth and disease resistance.
As you explore deeper into soil microbiology, you'll appreciate the complex web of life beneath our feet. This fascinating field continues to reveal insights into the critical role microorganisms play in maintaining the health and productivity of our soils.
Aquatic Microbiology
Just as soil teems with microbial life, aquatic environments host a intriguing diversity of microorganisms. You'll find them in every drop of water, from the tiniest puddle to the vast expanse of the ocean. These aquatic microbes play crucial roles in nutrient cycling, decomposition, and supporting the food web.
In freshwater habitats like lakes, rivers, and streams, you'll encounter bacteria, algae, protozoa, and fungi. Cyanobacteria, also known as blue-green algae, are particularly important as they perform photosynthesis and contribute to primary production. Some aquatic microbes break down organic matter, while others establish symbiotic relationships with larger organisms.
Marine environments present unique challenges and opportunities for microbes. Salinity, pressure, and nutrient availability vary greatly, shaping microbial communities. In the sunlit upper layers, photosynthetic microbes thrive, forming the base of the marine food chain. As you descend into the depths, chemosynthetic bacteria harness chemical energy from hydrothermal vents and cold seeps.
Aquatic microbes also have significant impacts on human activities. They can cause waterborne diseases, contaminate drinking water, and affect aquaculture. However, they also offer potential solutions, such as bioremediation of polluted waters and the development of novel compounds with pharmaceutical and industrial applications.
Studying aquatic microbiology reveals the hidden world beneath the surface, showing the intricate relationships and adaptations of these tiny life forms. As you explore this intriguing field, you'll gain a deeper appreciation for the critical roles microbes play in maintaining the delicate balance of aquatic ecosystems.
Food Microbiology
Immerse yourself in the fascinating world of food microbiology, where microbes reign supreme in shaping the flavors, textures, and safety of what we eat. From the beneficial bacteria that ferment your favorite cheeses and yogurts to the dangerous pathogens that can cause foodborne illnesses, microorganisms play an essential role in the food industry. You'll discover how these tiny beings can make or break the quality and shelf life of various food products.
Dive into the processes of food spoilage and learn how to prevent it by understanding the growth requirements of different microbes. Explore the principles of food preservation techniques, such as pasteurization, canning, and drying, which aim to control microbial growth and extend the shelf life of foods. You'll also uncover the secrets behind the production of fermented foods, like sourdough bread, kimchi, and sauerkraut, where beneficial microbes work their magic to create unique flavors and textures.
Food safety is a vital aspect of food microbiology, and you'll learn about the common foodborne pathogens, such as Salmonella, E. coli, and Listeria, and how to prevent their growth and contamination in food processing environments. By understanding the principles of food microbiology, you'll be better equipped to guarantee the safety and quality of the food you consume and produce.
Industrial Microbiology
Explore the domain of industrial microbiology, where microorganisms are harnessed for their incredible abilities to produce valuable products on a large scale. You'll discover how these tiny organisms, often invisible to the naked eye, play a vital role in manufacturing everything from food and beverages to pharmaceuticals and biofuels. Industrial microbiologists carefully select and refine microbial strains to maximize their efficiency and productivity in controlled environments called bioreactors or fermenters.
You'll find that bacteria, yeasts, and molds are the most commonly used microorganisms in industrial settings. They're employed in the production of antibiotics, enzymes, amino acids, and various other bioactive compounds. The fermentation process, which has been used for centuries to make bread, beer, and wine, is now applied to create a wide array of products, including organic acids, vitamins, and even bioplastics.
As you investigate deeper into industrial microbiology, you'll learn about the importance of maintaining suitable growth conditions for these microorganisms, such as temperature, pH, and nutrient availability. You'll also encounter the challenges of scaling up production from laboratory to industrial levels while ensuring consistent quality and safety. With advances in genetic engineering and synthetic biology, industrial microbiologists are now able to modify microbial genomes to enhance their capabilities and create novel products, opening up exciting new possibilities for the future of biotechnology.
Pharmaceutical Microbiology
As you plunge into the domain of pharmaceutical microbiology, you'll discover the vital role microorganisms play in the development, production, and quality control of medicines. Pharmaceutical microbiologists harness the power of bacteria, fungi, and other microbes to create life-saving drugs, such as antibiotics, vaccines, and biopharmaceuticals. They also work tirelessly to prevent contamination and guarantee the safety and efficacy of pharmaceutical products.
In the field of drug discovery, you'll find microorganisms serving as invaluable sources of novel compounds with therapeutic potential. Through processes like fermentation and genetic engineering, pharmaceutical microbiologists optimize the production of these compounds, scaling up from lab to industrial levels. They also employ sophisticated techniques, such as high-throughput screening and metagenomics, to identify new drug candidates.
Quality control is another essential aspect of pharmaceutical microbiology. You'll learn about the stringent testing methods used to detect and eliminate microbial contaminants in raw materials, manufacturing environments, and finished products. Sterility testing, endotoxin testing, and antimicrobial effectiveness testing are just a few examples of the rigorous procedures in place to assure the purity and stability of medicines.
As you explore this fascinating field, you'll appreciate the immense impact pharmaceutical microbiology has on global health. From combating infectious diseases to advancing personalized medicine, the work of pharmaceutical microbiologists is at the forefront of medical innovation. So, get ready to dive deep into the microscopic world and uncover the secrets that could revolutionize healthcare as we understand it.
Microbial Biotechnology
Get ready to plunge into the enthralling world of microbial biotechnology, where tiny organisms make a colossal impact. In this field, you'll discover how microbes are harnessed to create products and services that benefit humanity. From pharmaceuticals to food production, environmental cleanup to biofuels, microbial biotechnology is a game-changer.
You'll be amazed by the diverse applications of microbial biotechnology. Genetically engineered bacteria produce insulin for diabetics, while others synthesize enzymes for detergents and food processing. Microbes are used to ferment foods like yogurt, cheese, and soy sauce, enhancing flavor and nutrition. They're even employed in bioremediation to break down pollutants and clean up oil spills.
As you explore microbial biotechnology, you'll learn about cutting-edge techniques like metabolic engineering, where microbes are modified to optimize product yield and purity. You'll encounter concepts like bioreactors, where microbes are cultivated under controlled conditions to maximize efficiency. You'll also discover the role of microbial biotechnology in developing sustainable alternatives to fossil fuels, such as bioethanol and biogas.
Microbial biotechnology is a rapidly evolving field, with new breakthroughs and applications emerging regularly. As you investigate further, you'll appreciate the incredible potential of these microscopic marvels and their profound impact on our lives. So, get set to initiate a fascinating journey into the world of microbial biotechnology, where innovation and sustainability go hand in hand.
Microbial Pathogenesis
While microbial biotechnology harnesses the power of microbes for beneficial purposes, it's important to recognize that some microorganisms can cause harm. Microbial pathogenesis is the process by which microbes cause disease in their hosts. Pathogens have evolved various strategies to invade, survive, and multiply within their hosts, leading to infection and disease.
To understand microbial pathogenesis, you need to be familiar with the different types of pathogens, such as bacteria, viruses, fungi, and parasites. Each type has its own unique mechanisms for causing disease. For example, some bacteria produce toxins that damage host tissues, while others invade and multiply within host cells.
Pathogenic microbes can enter the body through various routes, such as the respiratory tract, digestive system, or skin. Once inside, they must overcome the host's immune defenses to establish an infection. Some pathogens have evolved ways to evade or suppress the immune response, allowing them to persist and spread within the body.
The severity of microbial diseases can vary depending on factors such as the virulence of the pathogen, the dose of the infectious agent, and the immune status of the host. Some infections may be mild and self-limiting, while others can be severe and life-threatening.
Understanding microbial pathogenesis is essential for developing effective strategies to prevent, diagnose, and treat infectious diseases. This includes the development of vaccines, antibiotics, and other antimicrobial agents. By studying the mechanisms of microbial pathogenesis, scientists can identify new targets for therapeutic interventions and develop more effective ways to combat infectious diseases.
Bacterial Infections
Bacterial infections are one of the most common types of microbial diseases. They occur when harmful bacteria enter your body, multiply, and cause damage to tissues or disrupt normal functions. These infections can range from mild to severe and can affect various parts of your body, such as your skin, respiratory system, urinary tract, or bloodstream.
To understand bacterial infections, you need to know that not all bacteria are harmful. In fact, many bacteria are beneficial and even indispensable for your health. However, when pathogenic bacteria invade your body, they can cause infections by releasing toxins, damaging cells, or triggering an immune response.
Common bacterial infections include strep throat, pneumonia, urinary tract infections, and food poisoning. Symptoms can vary depending on the type of infection and the affected area but may include fever, chills, swelling, redness, pain, or discharge.
Treatment for bacterial infections often involves antibiotics, which are drugs that kill or stop the growth of bacteria. It's essential to follow your healthcare provider's instructions and complete the entire course of antibiotics, even if you start feeling better. Failing to do so can lead to antibiotic resistance, making it harder to treat future infections.
To prevent bacterial infections, practice good hygiene, such as washing your hands regularly, cleaning and disinfecting surfaces, and handling food safely. Staying up to date with vaccinations can also help protect you against certain bacterial diseases.
Viral Infections
Viral infections are another major category of microbial diseases. They're caused by viruses, which are tiny infectious agents that can only reproduce inside living host cells. Unlike bacteria, viruses aren't alive in the traditional sense. They consist of genetic material (DNA or RNA) wrapped in a protein coat. When a virus enters your body, it invades your cells and hijacks their machinery to make copies of itself. This process damages or kills the infected cells, leading to various symptoms and illnesses.
Viral infections can affect any part of your body, from your skin and respiratory system to your liver and brain. Common examples include the flu, common cold, measles, mumps, chickenpox, and genital herpes. Some viral infections, like HIV and certain types of hepatitis, can become chronic and lead to serious complications if left untreated.
Unlike bacterial infections, viral infections generally don't respond to antibiotics. Treatment usually focuses on relieving symptoms while your immune system fights off the virus. However, antiviral medications are available for some infections, such as influenza and HIV. Additionally, vaccines can prevent many viral diseases, including measles, mumps, rubella, and hepatitis A and B.
To reduce your risk of viral infections, practice good hygiene, such as washing your hands frequently, avoiding close contact with sick people, and disinfecting contaminated surfaces. Strengthening your immune system through a healthy diet, regular exercise, and adequate sleep can also help your body fight off viral invaders more effectively.
Fungal Infections
Fungal infections are the third main type of microbial disease you can contract. They're caused by various fungi, which are eukaryotic organisms distinct from bacteria and viruses. Fungi can be unicellular, like yeasts, or multicellular, like molds and mushrooms. Many fungal species are harmless or even beneficial, but some can cause infections when they invade your body, especially if you have a weakened immune system.
Common fungal infections include athlete's foot, jock itch, and ringworm, which affect your skin, nails, or hair. These infections are usually superficial and can be treated with over-the-counter antifungal creams or powders. However, if left untreated, they can spread and cause more severe problems.
More serious fungal infections can affect your internal organs, such as your lungs or brain. These infections are often opportunistic, meaning they take advantage of a compromised immune system. Examples include aspergillosis, candidiasis, and cryptococcosis. Symptoms can range from fever and cough to meningitis and seizures. Treatment typically involves oral or intravenous antifungal medications and addressing any underlying health issues.
To prevent fungal infections, keep your skin clean and dry, avoid sharing personal items like towels or combs, and wear shoes in public showers or locker rooms. If you have a weakened immune system, take extra precautions to avoid exposure to fungal spores, and consult your doctor if you suspect an infection.
Protozoan Infections
Protozoan infections round out the four main types of microbial diseases. While you may not hear about them as often as bacterial or viral illnesses, they can still wreak havoc on your health. Protozoa are single-celled eukaryotic organisms, and some species are parasitic, meaning they live and feed off other organisms, including humans. They're often spread through contaminated water or food, or by insect vectors like mosquitoes.
Some common protozoan infections include malaria, caused by Plasmodium species and transmitted by Anopheles mosquitoes; giardiasis, resulting from Giardia lamblia and often contracted from untreated water; and toxoplasmosis, due to Toxoplasma gondii, which you can get from undercooked meat or exposure to infected cat feces. Cryptosporidiosis, amebiasis, and trichomoniasis are a few others to watch out for.
Symptoms vary depending on the specific infection but often include gastrointestinal issues like diarrhea, abdominal pain, and nausea. Some protozoan diseases, particularly malaria, can be life-threatening if left untreated. Fortunately, antiparasitic medications are available to combat these infections. To prevent them in the first place, be cautious about water sources, practice good hygiene and safe sex, and cook your food thoroughly. If you suspect you've contracted a protozoan infection, don't hesitate to see your doctor for proper diagnosis and treatment. These tiny parasites pack a punch, but with the right knowledge and precautions, you can keep them from causing you harm.
Antimicrobial Resistance
As we've explored the various microbial threats to human health, it is vital to understand a growing challenge in treating these infections: antimicrobial resistance. This phenomenon occurs when microorganisms, such as bacteria, viruses, fungi, and parasites, develop the ability to survive exposure to antimicrobial drugs that were once effective in treating them. The misuse and overuse of antimicrobials have accelerated the development of resistant strains, making it harder to treat common infections.
You should be aware of the consequences of antimicrobial resistance, which include prolonged illness, increased healthcare costs, and a higher risk of complications and death. As more microbes become resistant to multiple drugs, we face the possibility of a post-antibiotic era where even minor infections could become life-threatening.
To combat antimicrobial resistance, it is important that you use antibiotics responsibly. Only take them when prescribed by a healthcare professional, and always complete the full course of treatment, even if you start feeling better. Don't share or use leftover antibiotics, and practice good hygiene to prevent the spread of infections.
Healthcare professionals, policymakers, and researchers must also work together to develop new antimicrobial drugs, improve diagnostic tools, and implement effective infection control measures. By understanding the gravity of antimicrobial resistance and taking action to address it, we can preserve the effectiveness of these life-saving drugs for future generations.
Microbial Identification
Identifying the culprit behind an infection is essential for selecting the most effective treatment. You'll find that microbial identification relies on various techniques to pinpoint the microbe responsible. These methods include microscopy, staining, biochemical tests, and molecular diagnostics. Microscopy lets you observe the microbe's size, shape, and structure. Staining techniques, such as Gram staining, help differentiate between bacterial groups based on their cell wall composition. Biochemical tests assess the microbe's metabolic capabilities and enzymatic reactions, providing clues to its identity. Molecular diagnostics, like PCR and DNA sequencing, offer more precise identification by analyzing the microbe's genetic material.
To identify a microbe, you'll typically start with a clinical sample, such as blood, urine, or wound swab. The sample is cultured on specific media to isolate the microbe. Once isolated, you'll perform a series of tests to narrow down the possibilities. The results are then compared to known microbial profiles to determine the most likely culprit. Accurate identification is essential for selecting targeted antimicrobial therapy and avoiding the use of broad-spectrum antibiotics that can contribute to antimicrobial resistance.
Advances in technology have revolutionized microbial identification. Automated systems, like MALDI-TOF mass spectrometry, can rapidly identify microbes based on their unique protein profiles. Whole genome sequencing provides even greater resolution, allowing for strain-level identification and tracking of outbreaks. As these technologies become more accessible, they'll streamline the identification process and improve patient outcomes by enabling faster, more targeted treatment decisions.
Staining Techniques
Staining techniques are a microbiologist's secret weapons for visualizing and differentiating microbes. They allow you to enhance the contrast between the microorganism and its background, making it easier to observe under a microscope. Different stains interact with specific components of the microbial cell, such as the cell wall, cytoplasm, or nucleic acids, providing valuable information about the microbe's structure and properties.
One of the most commonly used staining methods is the Gram stain, which differentiates bacteria into two major groups: Gram-positive and Gram-negative. This distinction is based on the composition of their cell walls and how they react to the staining procedure. Gram-positive bacteria retain the crystal violet dye and appear purple, while Gram-negative bacteria lose the dye and are counterstained with safranin, appearing pink.
Other staining techniques include the acid-fast stain, which identifies bacteria with waxy cell walls, such as Mycobacterium tuberculosis, and the endospore stain, which highlights bacterial endospores. Flagella staining is used to visualize the presence and arrangement of flagella, while capsule staining helps detect the polysaccharide capsule surrounding some bacteria.
Staining techniques aren't limited to bacteria; they can also be applied to fungi, parasites, and other microorganisms. By mastering these techniques, you'll gain a deeper understanding of the microbial world and be better equipped to identify and characterize the microbes you encounter in your studies and research.
Microscopy
Microscopy is your gateway to the unseen world of microorganisms. You'll use various types of microscopes to observe and study the morphology, structure, and behavior of microbes too small to be seen with the naked eye. The most common microscope you'll encounter is the light microscope, which uses visible light and a system of lenses to magnify specimens. Compound light microscopes allow you to view bacteria, fungi, and parasites at magnifications up to 1000x.
For higher magnifications and greater resolution, you'll turn to electron microscopes. Scanning electron microscopes (SEM) produce detailed 3D images of a specimen's surface, while transmission electron microscopes (TEM) provide 2D cross-sectional views of ultra-thin samples. These powerful tools let you explore the intricate details of viruses, cell organelles, and macromolecular structures.
Fluorescence microscopy is another valuable technique in your arsenal. By staining specific molecules or structures with fluorescent dyes, you can visualize and track their presence, location, and interactions within living cells. Confocal microscopy takes this a step further, enabling you to capture high-resolution 3D images of fluorescently labeled samples.
As you investigate deeper into the microscopic domain, you'll appreciate the important role microscopy plays in unraveling the mysteries of microbial life. Mastering the use of these instruments will be essential to your success as a microbiologist, providing invaluable insights into the fascinating world of microbes.
Culture Methods
Growing microbes in the lab is both an art and a science. You'll need to provide the right nutrients, environmental conditions, and growth factors to successfully cultivate them. The most common method is using solid or liquid media. Solid media, like agar plates, allow you to isolate individual colonies and study their morphology. Liquid media, such as broths, are used for growing larger quantities of microbes.
When preparing media, you must guarantee sterility to avoid contamination. Aseptic technique is essential, involving sterilizing equipment and working in a laminar flow hood. You'll also need to select the appropriate media for the specific microbes you're targeting, considering factors like pH, osmolarity, and special nutrient requirements.
Once you've inoculated the media, you'll incubate the cultures under ideal conditions for growth, which varies among microbial species. Temperature, atmosphere (aerobic or anaerobic), and incubation time are key factors. After incubation, you'll examine the cultures for growth and perform further tests to identify and characterize the microbes.
Some microbes are fastidious and require special culture techniques. These include using selective media to inhibit unwanted growth, enrichment media to promote growth of specific microbes, or cell culture for viruses. Preserving microbial cultures is also important for long-term storage and future use.
Mastering culture methods takes practice and attention to detail. By providing the right conditions and using proper techniques, you'll access the fascinating world of microbial growth and diversity in your lab.
Biochemical Tests
Once you've grown your microbes, it's time to figure out what they are. That's where biochemical tests come in. They're a set of procedures that help you identify the specific species or strain of bacteria you're dealing with.
These tests work by detecting the presence or absence of certain enzymes, metabolic pathways, or other biochemical characteristics unique to different microorganisms. For example, the catalase test checks if bacteria produce the enzyme catalase, which breaks down hydrogen peroxide. If bubbles form when you add hydrogen peroxide to a bacterial colony, it's catalase-positive.
Other common biochemical tests include the oxidase test, which detects cytochrome c oxidase, and the indole test, which checks for the ability to break down tryptophan. Carbohydrate fermentation tests assess if bacteria can ferment specific sugars, while the urease test looks for the enzyme that hydrolyzes urea.
To perform these tests, you'll typically inoculate a small amount of your bacterial culture into a test medium containing the necessary reagents. After incubation, you'll observe any color changes, gas production, or other visible reactions that indicate a positive or negative result.
Molecular Diagnostics
While biochemical tests are a mainstay in microbiology labs, molecular diagnostics take pathogen identification to the next level. They're based on detecting specific DNA or RNA sequences unique to a particular microorganism. Polymerase chain reaction (PCR) is a common technique that amplifies these genetic markers, allowing for rapid and accurate identification of pathogens, even in small quantities.
Another powerful tool is next-generation sequencing (NGS), which can sequence an organism's entire genome, providing thorough data for identification and characterization. NGS is particularly useful for identifying new or emerging pathogens and tracking disease outbreaks.
Microarrays, or DNA chips, allow for simultaneous testing of multiple genetic markers, making them efficient for screening a wide range of pathogens. They're often used in clinical settings to quickly identify infectious agents from patient samples.
In-situ hybridization techniques, such as fluorescence in-situ hybridization (FISH), use labeled probes to detect specific DNA or RNA sequences within intact cells or tissues. This allows for visualization of the spatial distribution of microorganisms.
Molecular diagnostics offer several advantages over traditional methods. They're highly sensitive, specific, and can provide results in a matter of hours. They can also detect pathogens that are difficult or impossible to culture. As technology advances, molecular methods will likely play an increasingly important role in microbiology, improving our ability to diagnose and manage infectious diseases.
Immunological Assays
Immunological assays harness the specificity of antibodies to detect and quantify microbial antigens or the host's immune response to infection. They're powerful tools in the microbiologist's arsenal for diagnosing infectious diseases, monitoring treatment efficacy, and understanding the interplay between pathogens and the immune system.
One commonly used immunological assay is the enzyme-linked immunosorbent assay (ELISA). In this technique, you immobilize the target antigen on a solid surface, such as a microtiter plate, and then add a specific antibody linked to an enzyme. If the antigen is present, the antibody binds to it, and the enzyme catalyzes a color change in a substrate, indicating a positive result. ELISA is highly sensitive and can detect even small amounts of antigen.
Another important assay is the western blot, which combines the specificity of antibodies with the resolving power of gel electrophoresis. In this method, you separate proteins from a microbial sample on a gel, transfer them to a membrane, and probe with specific antibodies. The antibodies bind to their target proteins, allowing you to identify and characterize specific microbial components.
Immunofluorescence assays use fluorescently labeled antibodies to visualize the location and distribution of microbial antigens in tissue samples or cell cultures. This technique provides valuable insights into the pathogenesis of infectious diseases and the host-pathogen interaction.
Microbial Control
Now that you've explored how immunological assays can detect and characterize microbes, let's shift our focus to controlling them. Microbial control is vital in various settings, from healthcare to food production, to prevent the spread of pathogens and guarantee public safety. There are several methods you can employ to control microbial growth and transmission.
Physical methods, such as heat sterilization, filtration, and radiation, can effectively eliminate or reduce microbial populations. Chemical methods involve the use of disinfectants, antiseptics, and antibiotics to kill or inhibit the growth of microorganisms. It is important to understand the proper application and limitations of each method to achieve the best results.
In healthcare settings, you'll encounter strict protocols for hand hygiene, personal protective equipment (PPE), and environmental cleaning to minimize the risk of nosocomial infections. Food production relies on Good Manufacturing Practices (GMP) and Hazard Analysis Critical Control Points (HACCP) to guarantee food safety and prevent foodborne illnesses.
Antimicrobial resistance is a growing concern, so it is vital to use antibiotics judiciously and follow guidelines for their appropriate use. By implementing effective microbial control measures, you can protect yourself, your community, and the environment from the adverse effects of harmful microorganisms. Understanding the principles and applications of microbial control is a fundamental aspect of microbiology that you'll continue to encounter in various fields.
Sterilization
Sterilization, an important aspect of microbial control, involves the complete elimination or destruction of all forms of microbial life, including vegetative cells, spores, and viruses. You can achieve sterilization through various methods, such as heat, radiation, filtration, and chemical agents. Heat sterilization is commonly used and can be accomplished by moist heat (autoclaving) or dry heat (hot air oven). Autoclaving involves exposing materials to saturated steam under pressure at 121°C for a specific duration, while dry heat sterilization uses hot air at 160-180°C for a longer period.
Radiation sterilization employs ionizing radiation, such as gamma rays or electron beams, to inactivate microorganisms. This method is often used for heat-sensitive materials or large-scale sterilization. Filtration sterilization removes microorganisms by passing liquids or gases through filters with pore sizes small enough to retain the microbes. This technique is suitable for heat-labile solutions or air sterilization.
Chemical sterilization involves using chemical agents, such as ethylene oxide gas or liquid sterilants like glutaraldehyde and hydrogen peroxide. These agents denature proteins and damage microbial DNA, rendering the microorganisms non-viable. It's vital to select the appropriate sterilization method based on the material to be sterilized, its heat and moisture sensitivity, and the required level of sterility. Proper monitoring and validation of sterilization processes guarantee their effectiveness and maintain a sterile environment in various settings, including healthcare, research, and industry.
Disinfection
Disinfection, an imperative process in microbial control, reduces the number of pathogenic microorganisms on surfaces or objects to a safe level, as determined by public health standards. Unlike sterilization, which eliminates all microorganisms, disinfection targets specific pathogens while allowing some non-harmful microbes to remain. You'll commonly find disinfectants used in healthcare settings, food processing facilities, and households to prevent the spread of infectious diseases.
When choosing a disinfectant, consider factors such as the target microorganisms, surface material compatibility, and potential health and environmental risks. Common disinfectants include chlorine compounds, alcohols, hydrogen peroxide, and quaternary ammonium compounds. Each has its advantages and limitations, so it's vital to select the appropriate disinfectant for your specific needs.
To guarantee effective disinfection, follow the manufacturer's instructions for dilution, application method, and contact time. Proper cleaning of surfaces before disinfection is also paramount, as organic matter can reduce the efficacy of some disinfectants. Always prioritize personal safety by wearing appropriate protective equipment and guaranteeing adequate ventilation during the disinfection process.
Regularly monitoring and evaluating your disinfection protocols is essential to maintain a safe and hygienic environment. Implement a thorough disinfection plan, train personnel on proper procedures, and periodically assess the effectiveness of your disinfection methods through microbiological testing or other validation techniques. By understanding the principles and best practices of disinfection, you'll be better equipped to control the spread of pathogens and protect public health.
Antimicrobial Agents
Let's explore the world of antimicrobial agents, an important component in the fight against pathogenic microorganisms. These substances can kill or inhibit the growth of bacteria, fungi, viruses, and parasites. They're classified based on their spectrum of activity, mechanism of action, and source. Broad-spectrum antimicrobials target a wide range of microbes, while narrow-spectrum ones focus on specific types. Some common mechanisms include cell wall synthesis inhibition, protein synthesis disruption, and nucleic acid synthesis interference.
You'll find antimicrobial agents in various forms, such as antibiotics, antifungals, antivirals, and antiparasitics. They can be derived from natural sources like plants and microorganisms or synthesized in laboratories. Penicillin, discovered by Alexander Fleming in 1928, was the first antibiotic and revolutionized medicine. However, the overuse and misuse of antimicrobials have led to the emergence of resistant strains, posing a significant global health threat.
To combat resistance, it's vital to use antimicrobials judiciously, follow proper dosing and duration, and develop new agents. Researchers are exploring novel strategies like combination therapies, phage therapy, and antimicrobial peptides. As a student of microbiology, understanding the principles and challenges of antimicrobial agents will equip you to contribute to this ongoing battle against microbial pathogens.
Vaccination
Vaccination, a cornerstone of public health, has saved countless lives by preventing infectious diseases. It works by introducing a harmless form or component of a pathogen into your body, stimulating your immune system to develop antibodies and memory cells specific to that pathogen. This prepares your immune system to swiftly recognize and fight off the actual disease-causing agent if you're ever exposed to it in the future.
Vaccines come in various types, such as live-attenuated, inactivated, toxoid, and conjugate vaccines. They're rigorously tested for safety and efficacy before being approved for widespread use. Vaccination schedules are designed to protect you at the most appropriate ages and with the best intervals between doses.
Herd immunity is an essential concept in vaccination. It occurs when a considerable portion of a population is vaccinated, reducing the likelihood of disease spread and indirectly protecting those who can't be vaccinated due to age, health conditions, or other factors.
Despite the overwhelming scientific evidence supporting vaccination's safety and effectiveness, misconceptions and misinformation have led to vaccine hesitancy in some communities. It's important to rely on credible sources, such as healthcare professionals and reputable scientific organizations, for accurate information about vaccines.
Vaccination has been instrumental in eradicating smallpox, nearly eliminating polio, and greatly reducing the incidence of diseases like measles, rubella, and diphtheria. By choosing to vaccinate, you're not only protecting yourself but also contributing to the well-being of your community.
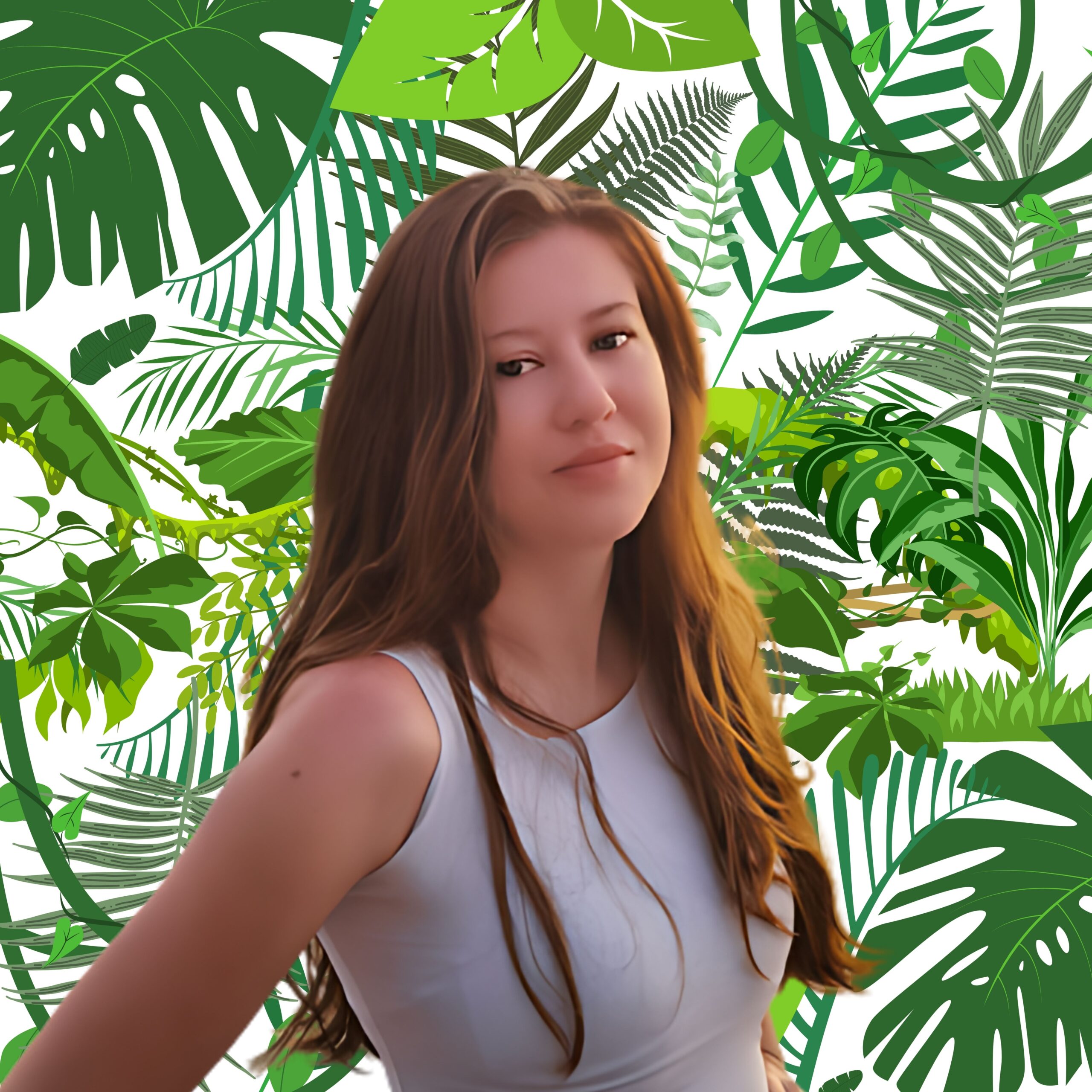
Erzsebet Frey (Eli Frey) is an ecologist and online entrepreneur with a Master of Science in Ecology from the University of Belgrade. Originally from Serbia, she has lived in Sri Lanka since 2017. Eli has worked internationally in countries like Oman, Brazil, Germany, and Sri Lanka. In 2018, she expanded into SEO and blogging, completing courses from UC Davis and Edinburgh. Eli has founded multiple websites focused on biology, ecology, environmental science, sustainable and simple living, and outdoor activities. She enjoys creating nature and simple living videos on YouTube and participates in speleology, diving, and hiking.