Bacterial cell walls are complex structures that provide shape, protection, and support. They're composed primarily of peptidoglycan, a mesh-like polymer of sugars and amino acids. In Gram-positive bacteria, you'll find a thick peptidoglycan layer with teichoic acids. Gram-negative bacteria have a thinner peptidoglycan layer sandwiched between inner and outer membranes, with lipopolysaccharides on the outer surface. These differences impact antibiotic effectiveness and bacterial survival. Cell wall components are vital targets for antibiotics and play roles in bacterial virulence. Understanding their composition is key to developing new treatments and diagnostic tools. Dive deeper to uncover the intricate world of bacterial cell walls.
Peptidoglycan Structure and Function
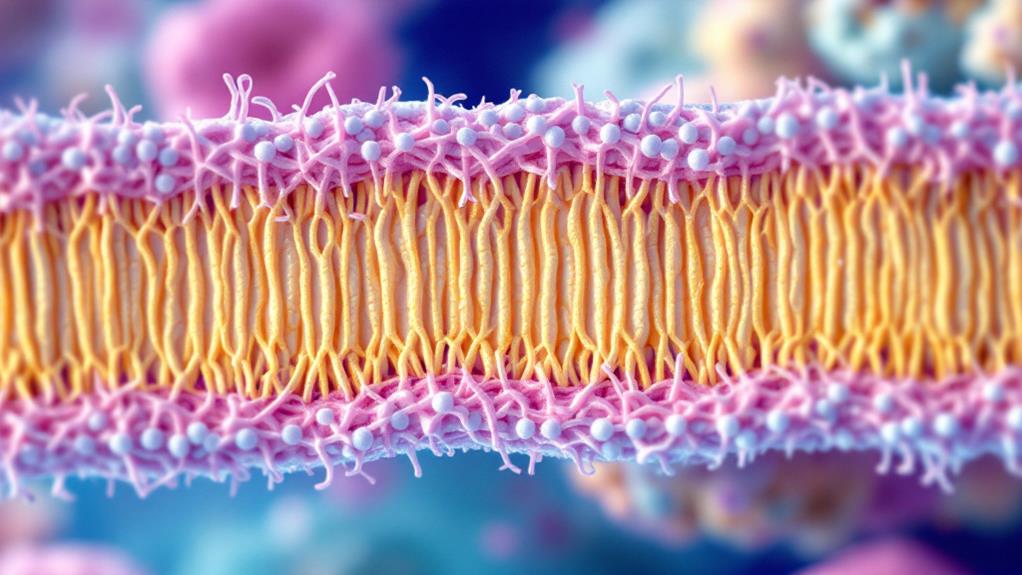
For decades, peptidoglycan has been recognized as an essential component of bacterial cell walls. This complex polymer forms a mesh-like structure that provides structural integrity and protection to bacterial cells. You'll find peptidoglycan in both Gram-positive and Gram-negative bacteria, though its thickness varies between these two groups.
Peptidoglycan consists of alternating N-acetylglucosamine (NAG) and N-acetylmuramic acid (NAM) sugar units, linked by β-1,4 glycosidic bonds. These sugar chains are cross-linked by short peptide bridges, creating a rigid, three-dimensional network. The composition of these peptide bridges can vary among different bacterial species, contributing to their unique cell wall characteristics.
The primary functions of peptidoglycan include maintaining cell shape and preventing osmotic lysis. It acts as a scaffold for other cell wall components and serves as an attachment point for various proteins and surface structures. You'll notice that peptidoglycan also plays a fundamental role in cell division, as it's involved in the formation of the septum during bacterial cell splitting.
Peptidoglycan synthesis is a complex process involving multiple enzymes. It's a target for many antibiotics, including penicillins and cephalosporins, which interfere with its assembly. Understanding the structure and function of peptidoglycan is essential for developing new antimicrobial strategies and combating antibiotic resistance.
When you study bacterial cell walls, you'll find that peptidoglycan's unique composition and properties make it an ideal marker for distinguishing between different bacterial types and a valuable tool in bacterial classification and identification.
Gram-Positive Cell Wall Architecture
While peptidoglycan is a common feature in bacterial cell walls, its arrangement and associated structures differ markedly between Gram-positive and Gram-negative bacteria. In Gram-positive bacteria, you'll find a thick, multilayered peptidoglycan structure that forms the outermost layer of the cell wall.
This peptidoglycan layer in Gram-positive bacteria is typically 20-80 nanometers thick, accounting for 30-70% of the cell wall mass. It's composed of numerous interconnected layers of peptidoglycan, providing substantial structural strength and rigidity to the cell. You'll notice that this thick layer is the primary reason Gram-positive bacteria retain the crystal violet dye during Gram staining.
Within the peptidoglycan matrix, you'll find other important components. Teichoic acids, which are polymers of glycerol or ribitol linked by phosphate groups, extend through the peptidoglycan layers and contribute to the cell's negative charge. There are two types: wall teichoic acids, which are covalently bonded to peptidoglycan, and lipoteichoic acids, which are anchored to the cell membrane.
You'll also encounter various surface proteins embedded in or attached to the peptidoglycan layer. These proteins serve diverse functions, including adhesion to host cells, nutrient acquisition, and evasion of host immune responses. Some Gram-positive bacteria may also have an additional layer called the S-layer, composed of protein or glycoprotein subunits, which sits atop the peptidoglycan.
Understanding this architecture is essential for developing antibiotics and comprehending bacterial pathogenesis, as the cell wall is often the target of both host defenses and medical interventions.
Gram-Negative Cell Wall Complexity
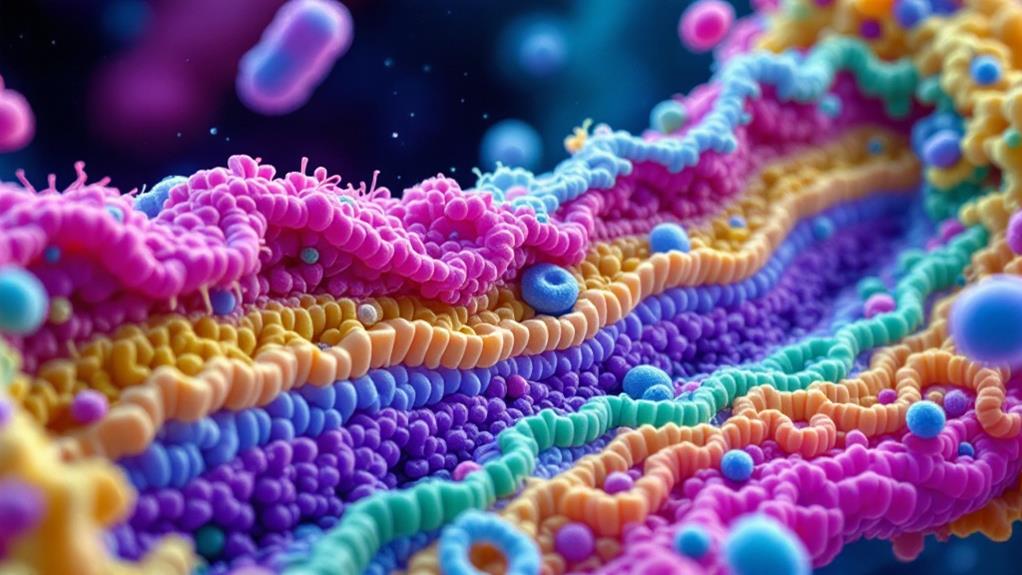
The complexity of Gram-negative cell walls stands in stark contrast to their Gram-positive counterparts. You'll find that Gram-negative bacteria possess a unique outer membrane, which adds an extra layer of protection and complexity to their cell wall structure.
This outer membrane is composed of lipopolysaccharides (LPS) on its exterior surface and phospholipids on its interior. The LPS molecules are vital for the bacteria's survival, as they help protect against host immune responses and certain antibiotics. You'll notice that the space between the outer membrane and the inner membrane is called the periplasmic space.
Within the periplasmic space, you'll find a thin layer of peptidoglycan. Unlike Gram-positive bacteria, this peptidoglycan layer is much thinner and doesn't provide as much structural support. Instead, the outer membrane takes on this role.
The inner membrane of Gram-negative bacteria is similar to that of Gram-positive bacteria, consisting of a phospholipid bilayer with embedded proteins. However, you'll observe that the overall structure is more complex due to the additional layers.
You'll also find specialized proteins called porins in the outer membrane. These proteins form channels that allow for the selective passage of small molecules, playing a vital role in nutrient uptake and waste removal.
Understanding the complexity of Gram-negative cell walls is essential, as it impacts their susceptibility to antibiotics and their interactions with host immune systems. This knowledge can help you develop more effective treatments for infections caused by Gram-negative bacteria.
Teichoic Acids in Cell Walls
Teichoic acids play an essential role in the structure and function of many bacterial cell walls, particularly in Gram-positive bacteria. These polymers are composed of glycerol phosphate, ribitol phosphate, or other sugar alcohols linked by phosphodiester bonds. You'll find two main types of teichoic acids in bacterial cell walls: wall teichoic acids (WTAs) and lipoteichoic acids (LTAs).
WTAs are covalently attached to the peptidoglycan layer, extending outward from the cell surface. They're often decorated with D-alanine or various sugars, which contribute to their diverse functions. LTAs, on the other hand, are anchored to the cell membrane and extend into the peptidoglycan layer.
These molecules serve several vital functions in bacterial cells. They help maintain cell shape and integrity by providing a rigid framework. Teichoic acids also play a role in regulating cell division and controlling autolytic enzymes. You'll find that they're involved in ion homeostasis, acting as a reservoir for cations like magnesium and calcium.
Teichoic acids contribute to bacterial pathogenicity by helping cells adhere to host tissues and evade immune responses. They can also interact with bacteriophages, either facilitating or preventing infection. In some cases, teichoic acids serve as receptors for certain antibiotics.
You should note that the composition and structure of teichoic acids can vary markedly between bacterial species. This variability contributes to the unique properties and behaviors of different bacteria. Understanding teichoic acids is vital for developing new antibiotics and strategies to combat bacterial infections.
Lipopolysaccharides and Outer Membranes
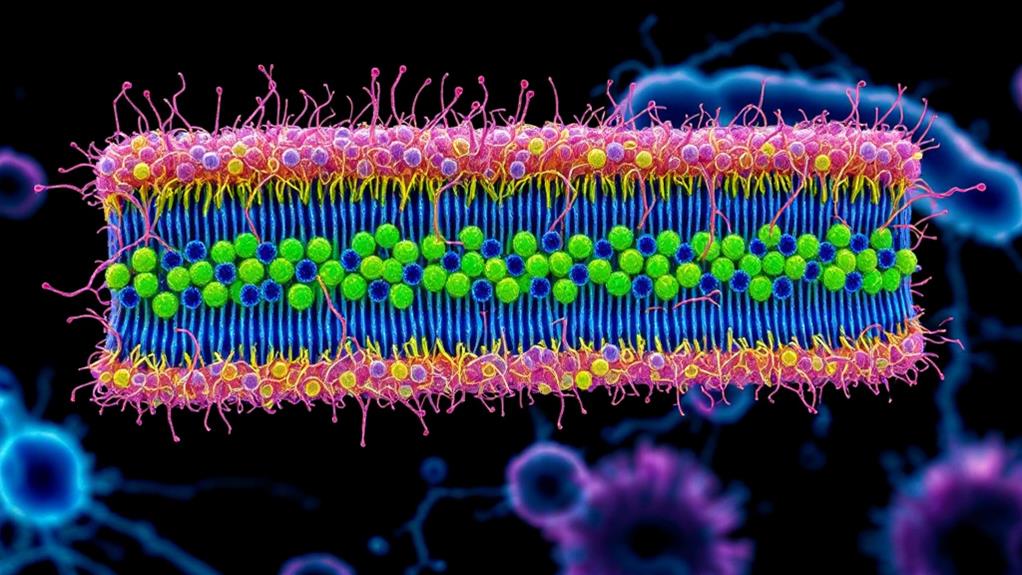
When you examine Gram-negative bacteria, you'll discover a unique outer membrane structure that's absent in their Gram-positive counterparts. This outer membrane is primarily composed of lipopolysaccharides (LPS), which play an essential role in the bacteria's survival and virulence.
Lipopolysaccharides consist of three main components: lipid A, core oligosaccharide, and O-antigen. Lipid A, the innermost part, anchors the LPS to the outer membrane and is responsible for the molecule's endotoxic properties. The core oligosaccharide connects lipid A to the O-antigen and contains unique sugar molecules. The O-antigen, a repeating oligosaccharide unit, extends outward from the bacterial surface and contributes to the cell's antigenic properties.
The outer membrane of Gram-negative bacteria serves as a protective barrier, regulating the entry of substances into the cell. It's asymmetrical, with the inner leaflet composed of phospholipids and the outer leaflet primarily made up of LPS. This arrangement creates a highly impermeable barrier that helps the bacteria resist various antibiotics and host immune responses.
You'll find that the outer membrane also contains proteins called porins, which form channels allowing the passage of small molecules. These porins are vital for nutrient uptake and waste excretion. Additionally, the outer membrane houses other proteins involved in cell adhesion, signaling, and virulence factor secretion.
Understanding the structure and function of lipopolysaccharides and outer membranes is fundamental in developing new antibiotics and vaccines targeting Gram-negative bacteria. Their unique composition makes them both a formidable defense mechanism and a potential weakness to exploit in medical treatments.
Cell Wall Synthesis Mechanisms
To understand bacterial resilience, you'll need to explore the intricate process of cell wall synthesis. Bacteria have developed sophisticated mechanisms to construct their cell walls, which are essential for survival and protection against environmental stresses.
The synthesis of peptidoglycan, a key component of bacterial cell walls, involves several steps. It begins in the cytoplasm, where precursor molecules are assembled. You'll find that UDP-N-acetylmuramic acid-pentapeptide and UDP-N-acetylglucosamine are the primary building blocks. These precursors are then transported across the cell membrane by lipid carriers.
Once on the outer side of the membrane, the precursors are polymerized into long chains by glycosyltransferases. These chains are then cross-linked by transpeptidases, forming a mesh-like structure that gives the cell wall its strength and rigidity.
In Gram-positive bacteria, you'll observe that teichoic acids are also incorporated into the cell wall during synthesis. These polymers contribute to the overall negative charge of the cell surface and play roles in ion homeostasis and antibiotic resistance.
For Gram-negative bacteria, you'll notice that the synthesis process includes the formation of the outer membrane. This involves the assembly and transport of lipopolysaccharides and outer membrane proteins.
The entire cell wall synthesis process is tightly regulated and coordinated with cell growth and division. Bacteria use various regulatory mechanisms, including two-component systems and cell division proteins, to guarantee proper cell wall formation. Understanding these mechanisms has led to the development of numerous antibiotics that target different stages of cell wall synthesis.
Antibiotic Targets in Cell Walls
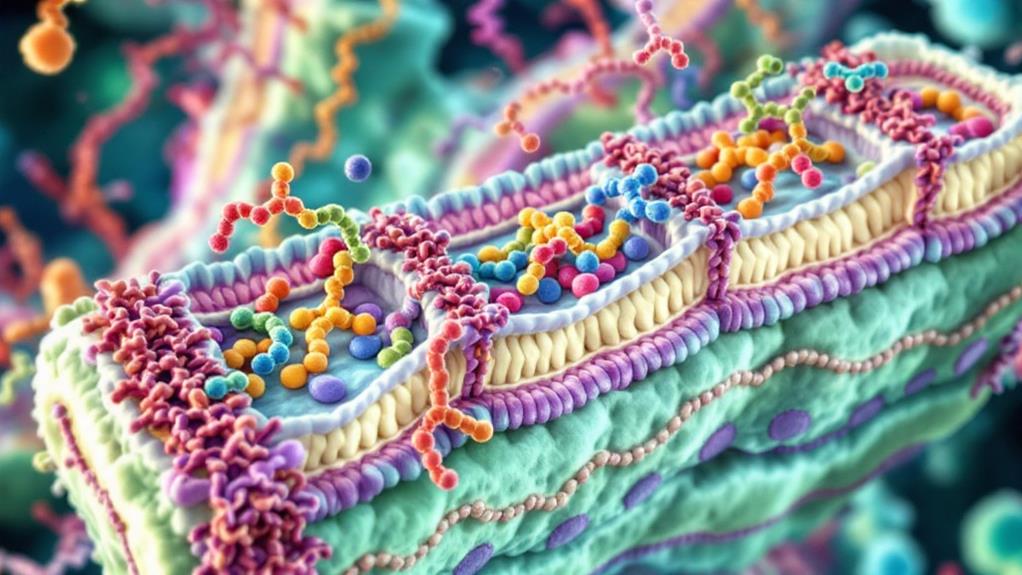
The bacterial cell wall presents several vital targets for antibiotic action. You'll find that many antibiotics exploit these targets to disrupt cell wall integrity and kill bacteria. One of the primary targets is the peptidoglycan layer, which is essential for maintaining cell shape and protecting against osmotic pressure.
Beta-lactam antibiotics, such as penicillin and cephalosporins, target the enzymes responsible for peptidoglycan cross-linking. They inhibit transpeptidases, also known as penicillin-binding proteins (PBPs), preventing the formation of strong peptide bonds between glycan strands. This weakens the cell wall, leading to bacterial lysis.
Another important target is the lipid II molecule, a precursor in peptidoglycan synthesis. Antibiotics like vancomycin bind to lipid II, blocking its incorporation into the growing cell wall. This interference disrupts the entire peptidoglycan assembly process.
You'll also find antibiotics that target earlier stages of cell wall synthesis. For example, fosfomycin inhibits the enzyme MurA, which catalyzes the first step in peptidoglycan formation. By doing so, it prevents the production of essential cell wall components.
Some antibiotics, like polymyxins, target the outer membrane of Gram-negative bacteria. They interact with lipopolysaccharides, disrupting membrane integrity and increasing permeability.
Understanding these antibiotic targets is imperative for developing new drugs and combating antibiotic resistance. As bacteria evolve mechanisms to resist existing antibiotics, researchers continue to explore novel ways to exploit cell wall vulnerabilities. This ongoing battle between antibiotics and bacteria underscores the importance of cell wall targets in microbial warfare.
Evolutionary Adaptations of Cell Walls
Bacterial cell walls have undergone remarkable adaptations throughout evolutionary history. You'll find that these adaptations have allowed bacteria to thrive in diverse environments and withstand various challenges.
One of the most significant evolutionary changes is the development of different cell wall structures in gram-positive and gram-negative bacteria. Gram-positive bacteria have evolved thick layers of peptidoglycan, providing enhanced structural integrity and protection against environmental stressors. In contrast, gram-negative bacteria have developed a thinner peptidoglycan layer but compensated with an additional outer membrane, offering increased resistance to certain antibiotics and host immune responses.
You'll notice that some bacteria have adapted their cell walls to extreme environments. For instance, thermophilic bacteria have evolved specialized lipids and proteins in their cell walls to maintain stability at high temperatures. Similarly, acidophilic bacteria have modified their cell wall composition to withstand low pH environments.
Another important adaptation is the evolution of cell wall-less bacteria, known as L-forms. These bacteria have lost their cell walls entirely, allowing them to evade certain antibiotics and immune responses. This adaptation demonstrates the remarkable plasticity of bacterial genomes.
Some bacteria have developed the ability to modify their cell wall composition in response to environmental cues. For example, certain species can alter their peptidoglycan structure to resist antibiotics or change their surface proteins to evade host immune recognition.
These evolutionary adaptations highlight the incredible diversity and resilience of bacterial cell walls, showcasing their importance in bacterial survival and pathogenicity.
Cell Wall Analysis Techniques
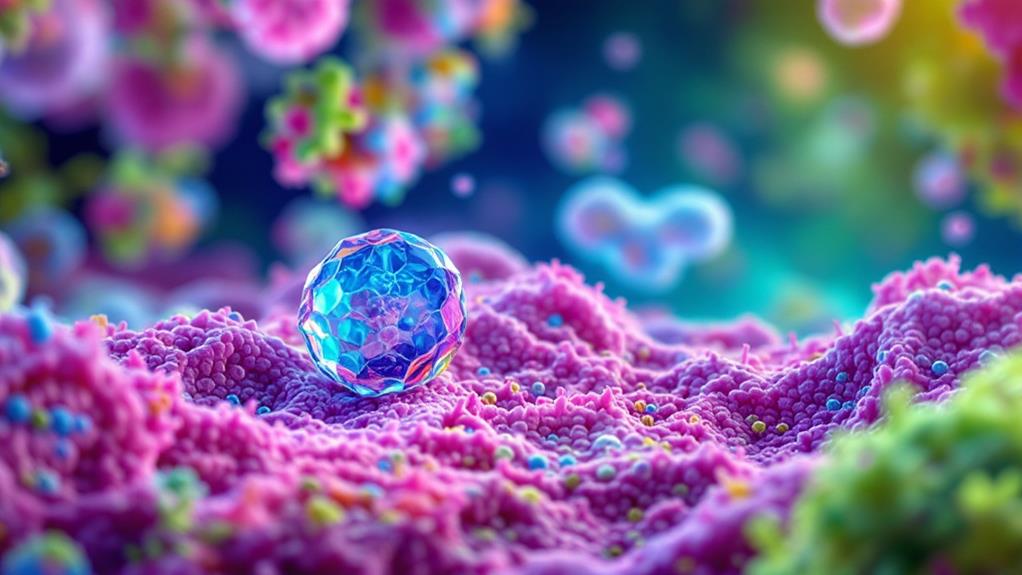
Scientists have developed numerous techniques to explore the complexities of bacterial cell walls. You'll find that these methods range from traditional microscopy to cutting-edge spectroscopic approaches.
Electron microscopy is a powerful tool you can use to visualize cell wall structures at high resolution. Transmission electron microscopy (TEM) allows you to examine thin sections of bacterial cells, revealing the layered organization of the cell wall. Scanning electron microscopy (SEM) provides detailed surface information, helping you understand the topography of the cell wall.
If you're interested in the chemical composition of cell walls, you'll want to employ techniques like gas chromatography-mass spectrometry (GC-MS) or high-performance liquid chromatography (HPLC). These methods enable you to identify and quantify specific components of the cell wall, such as peptidoglycan precursors or lipopolysaccharides.
For a more thorough analysis, you can turn to nuclear magnetic resonance (NMR) spectroscopy. This technique allows you to study the structure and dynamics of cell wall components in their native state. You'll gain insights into the molecular interactions within the cell wall.
X-ray crystallography is another valuable tool you can use to determine the three-dimensional structure of isolated cell wall components. This method provides atomic-level resolution, helping you understand the precise arrangement of molecules within the cell wall.
Lastly, you'll find that fluorescence microscopy techniques, such as super-resolution microscopy, are increasingly important. These methods allow you to visualize specific cell wall components in living bacteria, providing dynamic information about cell wall assembly and remodeling processes.
Medical Implications of Cell Walls
Delving into the medical implications of bacterial cell walls reveals their essential importance in both pathogenesis and treatment strategies. You'll find that these structures play a vital role in bacterial survival and virulence, making them prime targets for antibiotics and vaccine development.
The cell wall's composition directly affects a bacterium's ability to cause disease. Gram-positive bacteria, with their thick peptidoglycan layer, can withstand harsh environments and evade host immune responses. Gram-negative bacteria's outer membrane, containing lipopolysaccharides, can trigger severe inflammatory responses in hosts, leading to septic shock.
You'll notice that many antibiotics target cell wall synthesis. Penicillins and cephalosporins inhibit peptidoglycan cross-linking, while vancomycin interferes with peptidoglycan precursor formation. Understanding these mechanisms is vital for developing new antimicrobial agents to combat antibiotic resistance.
Cell wall components also serve as important antigens for vaccine development. You can see this in vaccines against pneumococcal diseases, which target capsular polysaccharides. Similarly, research into mycobacterial cell wall components aims to improve tuberculosis vaccines.
Diagnostically, cell wall characteristics help you identify bacterial species and guide treatment decisions. Techniques like Gram staining rely on cell wall properties to differentiate between bacterial types rapidly.
In personalized medicine, you'll find that understanding cell wall variations among bacterial strains can help predict antibiotic susceptibility and inform targeted therapies. This knowledge is particularly valuable in treating infections caused by multidrug-resistant bacteria.
As you explore further, you'll discover that ongoing research into bacterial cell walls continues to disclose new potential targets for therapeutic interventions and diagnostic tools.
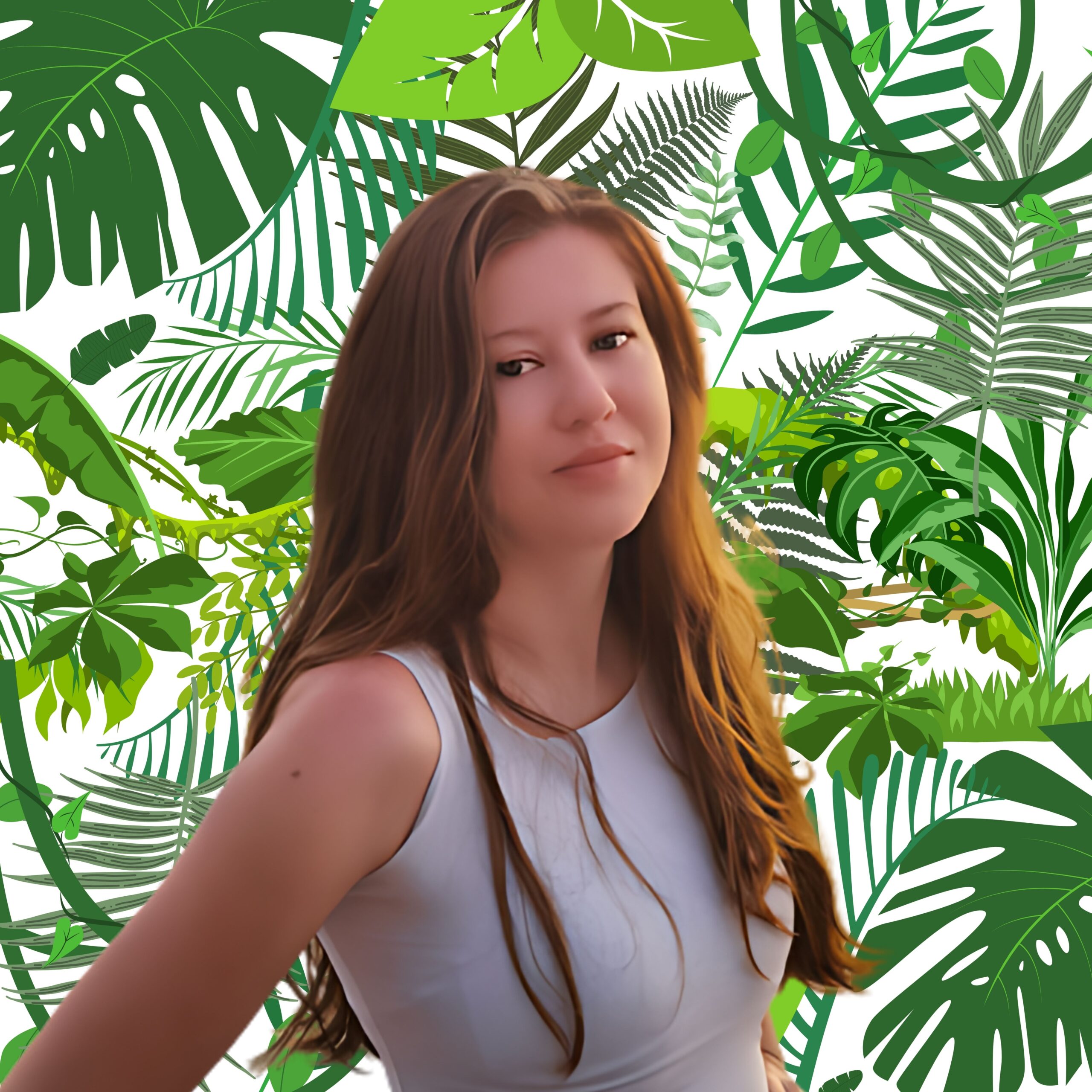
Erzsebet Frey (Eli Frey) is an ecologist and online entrepreneur with a Master of Science in Ecology from the University of Belgrade. Originally from Serbia, she has lived in Sri Lanka since 2017. Eli has worked internationally in countries like Oman, Brazil, Germany, and Sri Lanka. In 2018, she expanded into SEO and blogging, completing courses from UC Davis and Edinburgh. Eli has founded multiple websites focused on biology, ecology, environmental science, sustainable and simple living, and outdoor activities. She enjoys creating nature and simple living videos on YouTube and participates in speleology, diving, and hiking.